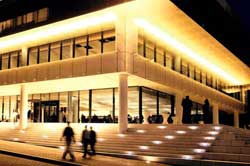
Overview
The focus of the 2007 Arctic Forum was: "Water in the Arctic: International Collaborations and Understanding Environmental Change."
The Arctic Forum was timed to coincide with a special program at the Embassy of Sweden on "Water and Environment," which offers reflections on global, national, regional and local perspectives regarding water. Forum sessions included a diverse and international range of perspectives on the state of knowledge of the hydrological cycle in the circumpolar Arctic, gaps in our knowledge, and research and policy opportunities and priorities. The Arctic Forum sessions highlighted international collaborations for the International Polar Year and beyond.
Presentations included information on:
- What are the changes being witnessed in the water cycle and how will these changes impact the physical, biological and social environments of the Arctic?
- How does the science of water and the arctic environment relate to public policy, and how can information be translated to decision makers?
- How can we forge international collaborations during IPY and beyond to address these exciting scientific challenges and opportunities?
- The 2007 Arctic Forum was co-chaired by Larry Hinzman, University of Alaska Fairbanks, and Gunhild Rosqvist, Stockholm University.
In addition to highlighting current research and policy issues relevant to the Arctic through the Arctic Forum, the ARCUS Annual Meeting serves to gather together members of the arctic research community, key agency personnel and policy makers, as well as ARCUS member institution representatives, board members, and staff.
A Congressional Science Briefing on Observing, Understanding, and Responding to Arctic Change was held on Tuesday, 22 May 2007.
2007 Arctic Forum Volume
The volume of abstracts from the 2007 Arctic Forum are available below in PDF format.
List of Abstracts
The Arctic Water Resources Vulnerability Index (AWRVI): A New Tool for Assessing Resilience to Change.
Lilian Alessa1, Andrew Kliskey2, Richard Lammers3, Christopher D. Arp4, Daniel M. White5, Larry D. Hinzman6
1Resilience and Adaptive Management Group, University of Alaska Anchorage, 3211 Providence Drive, Anchorage, AK, 99508, USA, Phone 907-786-1507, Fax 907-786-1314, afla [at] uaa.alaska.edu
2Resilience and Adaptive Management Group, University of Alaska Anchorage, 3211 Providence Drive, Anchorage, AK, 99508, USA, Phone 907-786-1136, Fax 907-786-4607, afadk [at] uaa.alaska.edu
3Water Systems Analysis Group, University of New Hampshire, Morse Hall 39 College Road, Room 211, Durham, NH, 03824, USA, Phone 603-862-4699, Fax 603-862-0587, richard.lammers [at] unh.edu
4Alaska Science Center, U.S. Geological Survey, 4230 University Drive, Suite 201, Anchorage, AK, 99508-4650, USA, Phone 907-786-7119, Fax 907-786-7150, carp [at] usgs.gov
5Institute of Northern Engineering, University of Alaska Fairbanks, 248 Duckering Building, PO Box 755900, Fairbanks, AK, 99775-5900, USA, Phone 907-474-6222, Fax 907-474-6866, ffdmw [at] uaf.edu
6International Arctic Research Center, University of Alaska Fairbanks, 930 Koyukuk Drive, PO Box 757340, Fairbanks, AK, 99775-7340, USA, Phone 907-474-7331, Fax 907-474-1578, ffldh [at] uaf.edu
To date no water vulnerability index exists for the Arctic, despite rapid changes in both climate and ecosystems. Several water indices have been developed in other parts of the world, but none has been developed, until now, to assess the resilience and vulnerability of fresh water resources in the Arctic.
The Arctic Water Resources Vulnerability Index (AWRVI) is a new and powerful on-the-ground tool for decision making which identifies where resilience and vulnerabilities exist in fresh water resources and how an action, such as development, may affect those resources. It works at the watershed scale, which is most relevant to the daily lives of arctic residents, and is based on the interactions of cumulative factors. Its Indicators include biophysical, sociocultural, economic and institutional components and it can be used by communities without extensive specialized training. Once AWRVI has been applied, a Resilience Score is determined for a community's water resources.
Using AWRVI, a community can assess the effects of environmental change on their social health and well-being. It combines both western and indigenous knowledge systems using the internet, oral traditions and existing data. It rapidly indicates where gaps in knowledge exist, and how urgent the need is to fill those gaps. The authors designed AWRVI to compile diverse information in a context that is relevant to a community's desires such that they can make informed decisions and adapt to change on their own terms.
The Arctic Ocean (Carbon Cycle) in a Changing Environment
Leif G. Anderson1
1Department of Chemistry, Göteborg University, Göteborg, SE-412 96, Sweden, Phone +46-31772-2774, Fax +46-31772-2785, leifand [at] chem.gu.se
Seawater enters the Arctic Ocean from both the Atlantic and Pacific Oceans with much of the surface water passing over very large Barents and Bering/Chukchi shelf seas. On its way north the surface water cools, which increases the solubility of gases and in summer significant primary production occurs that lowers pCO2. Both cooling and primary production promote a flux of CO2 from the atmosphere to the surface ocean. However, as much of the sea surface is covered by sea ice, at least during the winter season, gas exchange is hampered. One consequence of the seasonal ice cover is that the surface waters of the central Arctic Ocean are under-saturated with respect to CO2.
The Arctic Ocean is small compared to the global oceans and thus also the carbon fluxes are comparable small. However, in the inflowing shelf seas like the Barents Sea and Bering - Chukchi Seas primary production and air-sea fluxes of CO2 are per unity area many times the global average. The Pacific waters flowing into the Arctic Ocean through Bering Strait is through varying routes entering the North Atlantic, making the Arctic an important transport path between these two major global oceans. Another specific condition of the Arctic Ocean is the deep water formation that both transports dissolved organic and inorganic carbon from the surface ocean to the intermediate and deep waters, and also is an essential part in the sequestration of anthropogenic CO2. The formed sub-surface waters constitute the headwaters of the Meridional Overturning Circulation, spreading water to all global oceans.
At present the Arctic Ocean is undergoing rapid changes, e.g. decreasing sea ice coverage and increasing temperatures of the inflowing Atlantic Water, both impacting processes of importance for C-fluxes. Furthermore, the processes behind the deep water formation are sensitive to environmental change, which potentially could considerably alter these sinks. Especially since the formation of sea ice has been postulated to promote uptake of CO2 from the atmosphere when the surface water is under-saturated because the addition of brine from growing sea ice to the seawater provides an efficient means to transport enriched surface water to depth.
Furthermore, vast amounts of gas hydrates have been documented in association with thick arctic permafrost occurrences found beneath land areas and some shallow water areas of the continental shelf, as well as beneath deeper water areas of the Arctic Ocean. Because gas hydrates dissociate in response to warming temperature it have been speculated that methane from dissociating gas hydrate deposits could be released in a warming arctic climate.
Circumpolar Arctic Mammals and Consequences of Climate Change
Anders Angerbjörn1, Love Dalén2
1Department of Zoology, Stockholm University, Stockholm, SE-106 91, Sweden, Phone +46-816-4035, Fax +46-816-7715, angerbj [at] zoologi.su.se
2Centro Mixto UCM-ISCIII, c/ Sinesio Delgado 4-6, PabellÛn 14, Madrid, 28029, Spain, Phone +34664107127, love.dalen [at] zoologi.su.se
Species respond to an increased availability of habitat by increasing their distribution, for example at the end of the last glaciation. Hover, little is known about the opposite process, when the amount of habitat decreases. The hypothesis of habitat tracking predicts that species should be able to track both increases and decreases in habitat availability. The alternative hypothesis is that populations outside refugia become extinct during periods of unsuitable climate. To test this, we used ancient DNA techniques to examine genetic variation in the arctic fox (Alopex lagopus), whose geographic distribution was expanded during the last glaciation, and subsequently decreased during the Holocene. The southern Late Pleistocene populations have however, not contributed genetically to present day populations and did go extinct when the habitat shifted to the north. We don't know anything about the mechanisms. Today the Scandinavian arctic fox population is threatened to go extinct due decreasing amount of habitat, a decrease in food availability, i.e. lemming, and an increase in a temperate competitor, i.e. the red fox. These results provide new insights into how arctic species respond to climate change, and the mechanisms behind the extinction.
Wetland Carbon Stocks in Arctic Watersheds: We Might Know About How Much, But Where Is It?
David W. Beilman1
1Department of Geography, University of California Los Angeles, 1255 Bunche Hall, Los Angeles, CA, 90095, USA, Phone 541-760-6484, Fax 310-206-5976, dave.beilman [at] ucla.edu
The organic carbon (C) stocks contained in peat-accumulating wetland ecosystems were estimated for a southern region of the Mackenzie River Basin using high-resolution wetland map data, available soil C characteristic and peat depth datasets, and geostatistics. Peatlands cover 32% of the 25,119 km2 study area. The thickness of peat deposits measured at 203 sites was 2.5 m on average but as deep as 6 m, and highly variable between sites. Peat depths showed little relationship to terrain data within 1 to 5 km, but were spatially autocorrelated, and were generalized using Ordinary Kriging. Polygon-scale calculations and Monte Carlo simulations yielded a total peat C stock of 998 - 1010 Tg C that varied in mean C density (C mass per unit area) between 53 and 165 kg m^-2. This geostatistical approach showed as much as 10% more C than calculations using mean depths. This estimate was compared to an overlapping 7868 km^2 portion of the best independent peat C stock estimate for western continental North America, which revealed similar values for total peatland area, total C stock, and total C density. However, agreement was poor within ~875 km^2 grids owing to inconsistencies in wetland cover and little relationship in peat depth between estimates. The greatest disagreement in mean C density occurred in grids with the largest peatland cover, owing to the spatial coincidence of large cover and thick deposits in the high-resolution assessment. Existing peat C stock estimates in the southern Mackenzie Basin are likely of reasonable accuracy but, owing to uncertainties particularly in peat depth, we presently have limited information regarding the actual location of large stocks at scales as wide as several hundreds of square kilometers.
PolarTREC-Teachers and Researchers Exploring and Collaborating: Innovative Science Education from the Poles to the World
Katie J. Breen1, Janet Warburton2
1Project Manager, ARCUS, 3535 College Road, Suite 101, Fairbanks, AK, 99701, USA, Phone 907-474-1600, Fax 907-474-1604, breen [at] arcus.org
2Project Manager, ARCUS, 3535 College Road, Suite 101, Fairbanks, AK, 99709-3710, USA, Phone 907-474-1600, Fax 907-474-1604, warburton [at] arcus.org
PolarTREC-Teachers and Researchers Exploring and Collaborating is a three-year (2007-2009) teacher professional development program celebrating the International Polar Year (IPY) that will advance polar science education by bringing K-12 educators and polar researchers together in hands-on field experiences in the Arctic and Antarctic. PolarTREC builds on the strengths of the existing TREC program in the Arctic, an NSF supported program managed by the Arctic Research Consortium of the US (ARCUS), to embrace a wide range of activities occurring at both poles during and after IPY. PolarTREC will foster the integration of research and education to produce a legacy of long-term teacher-researcher collaborations, improved teacher content knowledge through experiences in scientific inquiry, and broad public interest and engagement in polar science and IPY.
PolarTREC will enable over fourty teachers to spend two to six weeks in the Arctic or Antarctic, working closely with researchers investigating a wide range of IPY science themed topics such as sea-ice dynamics, terrestrial ecology, marine biology, atmospheric chemistry, and long-term climate change. While in the field, teachers and researchers will communicate extensively with their colleagues, communities, and hundreds of students of all ages across the globe, using a variety of tools including satellite phones, online journals, podcasts and interactive "Live from IPY" calls and web-based seminars. The online outreach elements of the project convey these experiences to a broad audience far beyond the classrooms of the PolarTREC teachers. In addition to field research experiences, PolarTREC will support teacher professional development and a sustained community of teachers, scientists, and the public through workshops, Internet seminars, an e-mail listserve, and teacher peer groups.
To join the discovery, make global connections, and be part of the International Polar Year or, for more information, visit the PolarTREC website at:
http://www.polartrec.com
Or contact:
info [at] polartrec.com
907-474-1600
PolarTREC-Teachers and Researchers Exploring and Collaborating - Classroom Activities and Learning Resources
Katie J. Breen1, Janet Warburton2
1Project Manager, ARCUS, 3535 College Road, Suite 101, Fairbanks, AK, 99701, USA, Phone 907-474-1600, Fax 907-474-1604, breen [at] arcus.org
2Project Manager, ARCUS, 3535 College Road, Suite 101, Fairbanks, AK, 99709-3710, USA, Phone 907-474-1600, Fax 907-474-1604, warburton [at] arcus.org
This display highlights a variety of classroom activities that students completed in conjunction with various PolarTREC and TREC expeditions. Projects include artwork, songs, movies, research posters, and research papers. Contributions come from:
- Hanna High School, Brownsville, Texas
- Barrett Elementary School, Arlington, Virginia
- Concord High School, Concord, New Hampshire
- Horace Greeley High School, Chappaqua, New York
- Renfroe Middle School, Decatur, Georgia
For more information about PolarTREC see the PolarTREC abstract or visit the website at:
http://www.polartrec.com
Pan-Arctic Drainage Basin Monitoring: Current Status and Potential Significance for Assessment of Climate Change Impacts and Feedbacks
Arvid Bring1, Georgia Destouni2, Fredrik Hannerz3
1Department of Physical Geography and Quaternary Geology, Stockholm University, Stockholm, SE-106 91, Sweden, Phone +46 7 39 87 00 , arvid [at] bring.se
2Department of Physical Geography and Quaternary Geology, Stockholm University, Stockholm, -, SE-106 91, Sweden, Phone +46 8 16 47 85, georgia.destouni [at] natgeo.su.se
3Department of Physical Geography and Quaternary Geology, Stockholm University, Stockholm, -, SE-106 91, Sweden, Phone +46 8 16 48 86, Fax +46 8 16 47 94, fredrik.hannerz [at] natgeo.su.se
Access to reliable hydrologic data is of paramount importance for the accurate understanding of changes in the arctic hydrologic cycle, and is also vital to policymakers as a base for sound environmental decisions. Accessibility to such data is limited and continues to decline for some arctic areas, while little information exists on which data gaps are most critical. This study presents a quantitative assessment of openly available monitoring data for water discharge and chemistry in the pan-arctic drainage basin. Results indicate that there is significant disparity in the spatial and temporal distribution of accessible monitoring data, in particular for water chemistry monitoring. Additionally, there are systematic differences between the characteristics of monitored and unmonitored areas. These differences may limit the reliability of assessments of arctic water and solute flux changes under a warming climate. Arctic monitoring needs to be extended in certain areas, and data needs to be disseminated more efficiently, to fully enable characterization of the hydrologic variability and change in the region.
Arctic Atmospheric Hydrologic Cycle from Reanalyses and Climate Change
David Bromwich1, Sheng-Hung Wang2
1Byrd Polar Research Center , Ohio State University, Polar Meteorology Group, 1090 Carmack Road, Columbus, OH, 43210, USA, Phone 614-292-6692, Fax 614-292-4697, bromwich.1 [at] osu.edu
2Byrd Polar Research Center, Ohio State University, 108 Scott Hall, 1090 Carmack Road, Columbus, OH, 43210, USA, Phone 614-292-1060, Fax 614-292-4697, wang.446 [at] osu.edu
The atmospheric hydrologic cycle over the arctic basin has a significant impact on the mass balance of the sea ice cover. North Atlantic conditions are intimately related to the discharge from the Arctic Ocean through Fram Strait of sea ice and freshwater. The resulting variability in the North Atlantic thermohaline circulation can potentially impact global climate. However, the presence of a floating ice field prevents the collection of reliable measurements of atmospheric moisture and precipitation in the arctic region. In particular, measurements of solid precipitation are highly inaccurate when winds are strong, although statistical correction methods have been developed. Gauge-based measurements are also subject to blowing snow around the measurement sites. These limitations have led the exploration of the atmospheric moisture budget to derive estimates of precipitation minus evaporation/sublimation (P-E) from reanalysis data.
The two most widely used global reanalysis data sets are the collaborative effort of the National Centers for Environmental Prediction (NCEP) and the National Center for Atmospheric Research (NCAR) Reanalysis (NNR, 1948-present), and the European Centre for Medium-Range Weather Forecasts (ECMWF) 40-year Reanalysis (ERA-40, 1957-2002). A new global reanalysis data set from the Japan Meteorological Agency (JMA) and the Central Research Institute of Electric Power Industry (CRIEPI), the Japanese 25-year Reanalysis (JRA-25, 1979-present), is also available. These data sources are used to explore the long-term (1960-present) characteristics of the atmospheric moisture transport into the Arctic as a function of climate variability and change. In particular, an atmospheric contribution to the ongoing retreat of the arctic sea ice cover is sought.
The current shortcomings of global reanalyses in this data sparse region are discussed and a proposal is advanced to greatly improve our monitoring and understanding of this key climatic component.
ARCUS Internet Media Archive (IMA): A Resource for Outreach and Education
Tina M. Buxbaum1, Wendy K. Warnick2
1Project Manager, ARCUS, 3535 College Road, Suite 101, Fairbanks, AK, 99709-3710, USA, Phone 907-474-1600, Fax 907-474-1604, tina [at] arcus.org
2Executive Director, ARCUS, 3535 College Rd. Suite 101, Fairbanks, AK, 99701, USA, Phone 907-474-1600, Fax 907-4741604, arcus [at] arcus.org
The ARCUS Internet Media Archive (IMA) is a collection of photos, graphics, videos, and presentations that are shared through the Internet. It provides the arctic research community with a centralized location where images and video pertaining to polar research can be browsed and retrieved for a variety of uses. The IMA currently contains almost 5,000 publicly accessible photos, along with 360 video files, 260 audio files, and approximately 8,000 additional resources that are being prepared for public access.
The contents of this archive are organized by file type, photographer's name, event, or by organization, with each photo or file accompanied by information on content, contributor source, and usage requirements. All the files are keyworded and all information, including file name and description, is completely searchable.
ARCUS plans to continue to improve and expand the IMA with a particular focus on providing graphics depicting key arctic research results and findings as well as edited video archives of relevant scientific community meetings. To submit files or for more information and to view the ARCUS Internet Media Archive, please go to: http://media.arcus.org or email photo [at] arcus.org.
The Roles of Arctic Observatory Networks in Environmental Change Research and Monitoring
Terry V. Callaghan1, Margareta Johansson2, Torbjörn Johansson3, Craig E. Tweedie4, Mads C. Forchhammer5
1Abisko Scientific Research Station, Royal Swedish Academy of Sciences, Abisko, SE 981 07, Sweden, Phone +46-(0)-0980-40, terry_callaghan [at] btinternet.com
2Abisko Scientific Research Station, Lund University, Sølvegatan 12, Lund, SE-223 62, Sweden, Phone +46 46 222 42 6, Fax +46 46 222 40 1, scantran [at] ans.kiruna.se
3Lund University, Sovegatan 12, Lund, SE 981 07, Sweden, Phone +46 (0)46 222 3, Fax +46 (0)46 222 4, eusco [at] ans.kiruna.se
4Department of Biology, University of Texas at El Paso (UTEP), 500 West University Avenue, Biology Building Room 226, El Paso, TX, 79968-0513, USA, Phone 915-747-8448, Fax 915-747-5808, ctweedie [at] utep.edu
5Institute of Biology, University of Copenhagen, Universitetsparken 15, Copenhagen, DK-2100, Denmark, mcforchhammer [at] bi.ku.dk
Recent assessments of the accelerated global warming being experienced in the Arctic have highlighted uncertainties in our knowledge base while the US National Academies of Sciences and the Arctic Council of Ministers have independently identified the need for sustained and coordinated monitoring of the Arctic's environment. A workshop in 2004 identified the great potential that networks of Flagship Observatories could achieve by combining observation with research and outreach. SCANNET (Scandinavian and North European Network of Terrestrial Field Bases) is an example of an extensive network that combines both flagship sites and small field bases within wide climate, environmental and land use envelopes. Participating sites in SCANNET cover a range of mean annual temperatures from -10oC to +8oC, total annual precipitation from 200 to 2000 mm, vegetation zones from polar desert to grassland, and land use from occasional, past hunting to sheep farming. The geographical area also covers great variability in recent climate change from cooling to warming and the North Atlantic sites surround a region of potential profound importance to the world's ocean circulation and climate systems.
In this presentation, the diversity of contributions of SCANNET members to understanding environmental change will be demonstrated by using examples of two flagship observatories: the Abisko Research Station in the northern Swedish subarctic and the Zackenberg Research Station in the high Arctic of north-east Greenland. Long-term data series will be presented for the former showing complex 100-year changes in climate, environment and biota whereas comprehensive and integrated whole-ecosystem research and monitoring will be demonstrated for the latter. An example will be given of how a flagship observatory works with local stakeholders, the Sami, to enrich science and indigenous knowledge bases. Recently, SCANNET joined the US-led Circumarctic Environmental Observatories Network (CEON) that will unite North American, European and Russian networks and which is focusing initially on building web-based map servers for research and monitoring activities for observatories through out the Arctic.
Development of the Pan-Arctic Snowfall Reconstruction: New Land-based Solid Precipitation Estimates for 1940-1999
Jessie Cherry1, Bruno Tremblay2, Marc Stieglitz3, Stephen Dery4, Gavin Gong5
1International Arctic Research Center, University of Alaska Fairbanks, Arctic Regional Supercomputer Center, Fairbanks, AK, 99775-7335, USA, Phone 907-474-5730, jcherry [at] iarc.uaf.edu
2Department of Atmostpheric and Oceanic Sciences, McGill University, 805 Sherbrooke Street West, Montreal, QC, H3A 2K6, Canada
3School of Civil and Environmental Engineering, Georgia Institute of Technology, 790 Atlantic Avenue, Atlanta, GA, 30332-0355, USA, Phone 404-385-6530, Fax 404-385-1131, marc.stieglitz [at] ce.gatech.edu
4Environmental Science and Engineering Program, University of Northern British Columbia, 3333 University Way, Prince George, BC, V2N 4Z9, Canada, Phone 250-960-5193, Fax 250-960-5845, sdery [at] unbc.ca
5Department of Earth and Environmental Engineering, Columbia University, 500 West 120th Street, MC4711, New York, NY, 10027, USA, Phone 212-854-7287, gg2138 [at] columbia.edu
A new product, the Pan-Arctic Snowfall Reconstruction (PASR) is developed to address the problem of cold season precipitation gauge biases for the 1940-1999 period. The method used to create the PASR is different from methods used in other large-scale precipitation data products and has not previously been employed for estimating pan-arctic snowfall. The NASA Interannual-to-Seasonal Prediction Project Catchment Land Surface Model is used to reconstruct solid precipitation from observed snow depth and surface air temperatures. The method is tested at four stations in the United States and Canada where results are examined in depth. Reconstructed snowfall at Dease Lake, British Columbia and Barrow, Alaska is higher than gauge observations. Reconstructed snowfall at Regina, Saskatchewan and Minot, North Dakota is lower than gauge observations, probably because snow is transported by wind out of the Prairie region and enters the hydrometeorological cycle elsewhere. These results are similar to gauge biases estimated by a water budget approach. Reconstructed snowfall is consistently higher than snowfall from ECMWF Reanalysis-40 but does not have a consistent relationship with snowfall derived from the WMO Solid Precipitation Intercomparison Project correction algorithms. Advantages of the PASR approach include (1) the assimilation of snow depth observations captures blowing snow where it is deposited and (2) the modeling approach takes into account physical snowpack evolution. These advantages suggest the PASR product could be a valuable alternative to statistical gauge corrections and that arctic ground-based solid precipitation observing networks might emphasize snow depth measurements over gauges.
Intensification of the Arctic Hydrologic Cycle: Evidence from the Lena Basin, Siberia
Jessie Cherry1, Vladimir A. Alexeev2, Beate Liepert3, Pavel Groisman4, Vladimir Romanovsky5
1International Arctic Research Center, University of Alaska Fairbanks, P.O. Box 757335, Fairbanks, AK, 99775-7335, USA, Phone 907-474-5730, Fax 907-474-2643, jcherry [at] iarc.uaf.edu
2International Arctic Research Center, University of Alaska Fairbanks, PO Box 757340, Fairbanks, AK, 99775-7340, USA, Phone 907-474-6430, Fax 907-474-1578, valexeev [at] iarc.uaf.edu
3Lamont-Doherty Earth Observatory, Columbia University, PO Box 1000, 61 Rte. 9W, Palisades, NY, 10964, USA, Phone 845-365 8870, Fax 845-365 8154, liepert [at] ldeo.columbia.edu
4National Climate Data Center, National Oceanic and Atmospheric Administration, 151 Patton Avenue, Asheville, NC, 28801, USA, Phone 828-271-4347, pasha.groisman [at] noaa.gov
5Geophysical Institute, University of Alaska Fairbanks, PO Box 757320, Fairbanks, AK, 99775-7320, USA, Phone 907-474-7459, Fax 907-474-7290, ffver [at] uaf.edu
Strong positive climate feedbacks cause much of the Arctic to warm faster than the global average (IPCC, 2001; ACIA, 2004). The global hydrologic cycle is expected to accelerate in a warmer world because a warmer atmosphere can hold more water vapor. However, an important question that has not been adequately addressed is whether thawing of permafrost and deepening of the soil's active layer, which pulls moisture away from the surface into deeper reservoirs, will lead to a wetter or dryer arctic climate (Vorosmarty et al., 2001). There is an apparent paradox in the Arctic between increasing annual precipitation trends (ACIA, 2004), increasing occurrence of forest fires (Kasischke et al., 1999; Korovin and Zukkert, 2003), and the drying of surface lakes (Smith et al., 2005). Our investigation of hydroclimatological change in the Lena Basin (Russia) points to an increasingly wet Arctic. Though much of the near-surface air temperature (SAT) warming is occurring when the ground is covered by snow, increases in frozen precipitation are also contributing to warmer soil temperatures by increasing soil insulation. A deeper active layer caused by spring and summer warming holds more soil moisture and is leading to increasing potential evapotranspiration (shown in the model), increasing hydrologic baseflow (modeled and observed), and increasing summer nighttime cloudiness (observed). Changes in summer cloud types are suppressing warming during the days, but warming the nights significantly even during the polar day (Groisman et al., 1996). Earlier onset of snow cover in autumn traps the spring and summer warming, a trend that leads to further deepening of the active layer. These observed and modeled feedbacks describe an arctic hydroclimatological regime in which water storage and flow has increased and moved from the surface to the subsurface.
Consequences of Permafrost Thawing and Hydrological Changes for Landscape Scale Greenhouse Gas Exchange
Torben R. Christensen1
1GeoBiosphere Science Centre, Lund University, Physical Geography and Ecosystems Analysis, Sölvegatan 12, Lund, SE-223 62, Sweden, Phone +46 (0)46 22237, Fax +46 (0)46 22240, torben.christensen [at] nateko.lu.se
Northern wetlands are the largest single source of atmospheric methane. High northern latitudes host a large proportion of these wetlands often appearing as hot spots for atmospheric exchanges of both carbon dioxide and methane in composite landscapes. According to recent major international assessments such as the Arctic Climate Impact Assessment, the high northern latitudes will see significant climate change within coming decades. In addition to emissions of methane and potential substantial CO2 releases from stored soil organic carbon Arctic land and permafrost areas are influencing and interacting also in other ways with the climate system. Snow cover changes, permafrost thawing and the associated effects changing energy exchange is an obvious component of these interactions where also the vegetation composition and the position of the tree-line play an important role. Studies of interactions between the terrestrial biosphere and climate must pay attention to all these different and often interlinked processes. However, particular emphasis in studies of northern land-atmosphere interactions has been on measuring exchanges of carbon dioxide and methane. These greenhouse gases, in particular the latter, have the potential for strongly influencing the further development of climate. However, if the carbon budget of a northern landscape as a whole is to be studied the immediate atmospheric exchanges of trace gases are only one part of the story. Lateral transport of dissolved organic carbon and lake fluxes/sedimentation are factors that also are important for true catchment scale carbon and methane emission budgets and these are strongly influenced by permafrost dynamics. Here an attempt is made to evaluate the information available on all components of the carbon cycling including methane emissions in examples of composite northern landscapes, the Torneträsk region in northern Sweden and the Zackenberg valley in NE Greenland. Many components of the carbon cycling and methane emissions are associated with huge uncertainties and the budgets presented will merely be pointing these out as subjects of further studies rather than arguing final budgets for the landscapes are presented.
West Greenland Wildlife: Recent Caribou & Muskox Observations and Future Plans
Christine Cuyler1
1Department of Mammals and Birds, Greenland Institute of Natural Resources, PO Box 570, Nuuk, DK-3900, Greenland, Phone +299 36 12 40, Fax +299 36 12 12, chcu [at] natur.gl
Greenland is geographically isolated from North America, and has a limited diversity of terrestrial mammals. These few species will be subject to climate change without interference from invasive wild mammals moving north.
Models predict that by the end of the 21st century average temperature will rise 6-8°C in Northeast Greenland, following the expected retreat and reduction in sea ice cover, and 2-5°C in West Greenland. Furthermore, precipitation specifically in winter is predicted to increase 20-30%, with South and West Greenland experiencing greater precipitation changes than the rest of Greenland.
Today most Greenlanders live a modern city life far removed from that of their parents or grandparents. The recreational hunting of large herbivores, specifically caribou, provides an important bond to cultural traditions of the past, and for the commercial hunters an income. No holiday or special event would be complete without roast caribou on the menu, and although a relative newcomer to West Greenland, muskox has been steadily gaining favour. Recently, despite large herds, harvesting has been difficult. Local knowledge indicates that recent warmer temperatures have made access to hunting areas difficult or impossible, affected insect abundance and appear to have changed the amount of surface water and when it occurs. In addition, some caribou populations have changed their August-September distribution, and parasitic infestation appears to be increasing. To date documentation is lacking, but this is about to change.
NuukBasic is a new long-term study located around the Nation's capitol city, Nuuk, in sub arctic West Greenland. There are four research programs, MarineBasic, BioBasic, GeoBasic and ClimaBasic. Together they will produce knowledge about short and long-range variations in these arctic ecosystems. Understanding how climate change affects low and high arctic ecosystem structure and processes, will better define their relative vulnerability to expected changes.
BioBasic-Nuuk is an ecosystem-monitoring program starting this summer. The first full field season will be 2008. The project will be conducted in coordination with similar work already established at Zackenberg, which is the high arctic station in northeast Greenland (www.zackenberg.dk). Simultaneous observations of several terrestrial components, e.g., vegetation, soil fauna, birds, lake ecology, plant gas-flux (methane, CO2) and herbivores, will be related to local, regional and global climate change. Parameters will include, reproduction, distribution, phenology, species occurrence (plankton, arthropods, fish, birds, plants), and water and soil chemistry. The focus will be on a small fjord close to Nuuk, with a larger area to the north added to include caribou ecology. The Danish National Environmental Research Institute (DMU), Department of Arctic Environment, is responsible for the project with financing from the Danish Ministry of the Environment (MST).
Long-term monitoring in West Greenland's highly populated sub arctic region will increase understanding of the connection between human use of the animals and climate change's influence on these and their environment.
Changing Arctic Rivers
Stephen Dery1
1Environmental Science and Engineering Program, University of Northern British Columbia, 3333 University Way, Prince George, BC, V2N 4Z9, Canada, Phone 250-960-5193, Fax 250-960-5845, sdery [at] unbc.ca
This talk will present an overview of documented changes in arctic rivers during the 20th century, their implications and projections for future changes. To begin, a brief description of the pan-Arctic hydrological cycle and its climatology will be presented. Challenges of collecting hydrological data in harsh arctic conditions as well as other data issues will then be discussed. Changes in arctic rivers, both in Eurasia and North America, will then be presented, including observational evidence and attributions for change. Some implications of changing arctic rivers will follow and changes in arctic rivers projected for the 21st century will then be discussed. The talk will end with a summary and a list of research priorities concerning the impacts of climate change on arctic rivers.
Glacier Change in the Arctic: Important Consequences for Near Future Sea Level Rise
Andrew G. Fountain1
1Departments of Geology and Geography, Portland State University, 17 Cramer Hall, 1721 SW Broadway, Portland, OR, 97201, USA, Phone 503-725-3386, Fax 503-725-3025, andrew@pdxedu
Glaciers in the Arctic and sub-Arctic are rapidly changing in response to global warming. Although the spatial pattern of change varies around the hemisphere, over the past century the glaciers have been shrinking. The dominant influence on the glaciers is from rising air temperatures but local to regional variations in winter snowfall can buffer this influence. It appears that in recent years we have entered a new phase of glacier change. Not only have many alpine glaciers accelerated their shrinkage, but the Greenland Ice Sheet is now experiencing increased melt. In addition, the Greenland Ice Sheet appears to be dynamically responding to climate warming and is increasing ice flow into the ocean. The sum of ice melt and ice flow to the ocean is an important factor in current sea level rise and may accelerate in the near future further increasing sea level rise.
A Seasonally Ice Free Arctic?
Marika Holland1
1Climate and Global Dynamics Division, National Center for Atmospheric Research, PO Box 3000, Boulder, CO, 80307, USA, Phone 303-497-1734, Fax 303-497-1700, mholland [at] ucar.edu
Large and coordinated changes are occuring in the arctic climate system, including a significant retreat of the arctic summer sea ice. Climate models project that decreasing ice cover will continue into the foreseeable future and suggest that a seasonally ice-free Arctic could be realized within the next century. However, these models differ considerably in the rate and character of projected sea ice change. For example, a number of models exhibit abrupt retreat of the summer ice cover while others show more gradual change. Here, the mechanisms that drive rapid ice retreat are explored and a number of factors that influence whether climate models simulate rapid reductions in ice cover are addressed. This includes an analysis of simulated changes in arctic heat budgets and the role of forced versus natural variability in simulated sea ice change. To the extent possible, we discuss what this analysis suggests about how and when a seasonally ice-free Arctic might be realized.
Migration in the Arctic: Subsistence, Jobs, and Well-Being in Urban and Rural Communities
Lee Huskey1, Matt D. Berman2, Lance Howe3, Stephanie L. Martin4, Wayne Edwards5
1Department of Economics, University of Alaska Anchorage, 3211 Providence Drive, Anchorage, AK, 99508, USA, Phone 907.786.1905, Fax 907.7864115, aflh [at] uaa.alaska.edu
2Institute of Social and Economic Research, University of Alaska Anchorage, 3211 Providence Drive, Anchorage, AK, 99508, USA, Phone 907-786-5426, Fax 907-786-7739, auiser [at] uaa.alaska.edu
3Institute of Social and Economic Research, University of Alaska Anchorage, 3211 Providence Drive, Anchorage, AK, 99508, USA, elhowe [at] uaa.alaska.edu
4Institute of Social and Economic Research, University of Alaska Anchorage, 3211 Providence Drive, Anchorage, AK, 99508, USA, Phone 907-345-8130, Fax 907-345-8130, anslm1 [at] uaa.alaska.edu
5Department of Economics, University of Alaska Anchorage, 3211 Providence Drive, Anchorage, AK, 99508, USA, afwae [at] uaa.alaska.edu
Migration of the indigenous population of the Arctic is an important but little understood phenomenon. Migration affects the size and demographic structure of arctic communities. It is also an indicator of both community and individual well being. Migration behavior is influenced by and also affects the outcome of policy decisions at all levels of government.
The first step in understanding arctic migration is to describe its historic pattern. Relatively little is published about this pattern because access to much of the data that have been collected is legally restricted or difficult for researchers to obtain. Through support from the National Science Foundation and cooperation of the U.S. Census Center for Economic Studies, the North Slope Borough, and other organizations and individuals, we have gained access to much of these data.
This poster summarizes the patterns of movement of Alaska Natives in Alaska's arctic regions. Migration into and out of the region is described. Movement is traced both over time and along a hierarchy of places from village to regional center to the state's urban regions. Variation in migration rates across age, gender, and education groups is also examined. We compare migration rates for people with different characteristics and in different time periods since 1980, and summarize survey results of questions that provide insights into motivations for migration decisions. We evaluate our findings in light of arctic migration hypotheses discussed in previous literature and we generate new research questions and hypotheses about the determinants of migration patterns among the indigenous residents of arctic Alaska.
Hydrocarbons Biodegradation Potential In the Kola Bay (Barentz Sea) Littoral Area During Year In Relation with Macrophytes Growth
Vladimir V. Ilinskiy1, Inga V. Peretruchina2, Michail N. Semenenko3
1Hydrobiology Department, Moscow State Lomonosov University, Izmaylovskiy bulvar 34-25, Moscow, 105043, Russia, Phone 095-939-2573, Fax 095-939-4309, vladilin [at] interwave.ru
2Biological, Murmansk State Technological University, -, Russia
3Department of Chemistry, Moscow State Lomonosov University, Vorob'evi gori, Moscow, -, 119899, Russia, Phone +7-095-939-3845
Macrophytes usually produce some small amounts of hydrocarbons together with other organic substances during life cycle and algae leafs (talloms) can support a high microbial number and diversity. By these reasons its might be possible to suggest a higher hydrocarbon-oxidizing bacteria activity near macrophytes growth than far from its.
Hydrocarbon biodegradation potential (HBP) was investigated in Kola Bay littoral water near macrophytes growth, formed by Fucus and Laminaria species in main, and far off its. Samples were taken monthly during year.
HBP was measured as a sum of mineralization and biodegradation micro-bial processes with using 14C-octadecane (80 mcl/100 ml), water samples spiked with labeled hydrocarbon incubated then for 4 hours at in situ temperature.
A strong positive correlation (R = 0,86; a< 0,001) was observed between HBP and Kola Bay littoral water temperature during year with minimal HBP val-ues (lower than 200 ng l-1 h-1) during winter months December and January and maximal HBP values (near 300 ng l-1 h-1) during summer, in June and August. Kola Bay littoral water temperature during year varied from 1 to 10 degree ?. Summer and winter HBP values observed in Kola Bay, were near the same as maximal HBP values, which we observed early in Kandalaksha Bay (White Sea) near shore water during summer and winter periods.
Water samples taken from macrophytes growth demonstrated constantly more high (up to 20%) HBP values, than samples, taken far off macrophytes.
Our results show that macrophytes can stimulate microbial activities in relation to oil hydrocarbons during full year. We have no great differences between summer and winter HBP values and its possible to suggest that mesophilic hydrocarbon-oxidizing bacteria population, which presented in Kola Bay during summer, was replaced by psychrophilic bacteria population during winter period. As far as octadecane belong to n-alkanes, which is the most easily degrade by microorganisms, HBP values observed in Kola Bay during year may be near the maximal possible for oil hydrocarbons in this area.
Interannual Variability in the Arctic Ocean Freshwater Balance
Alexandra Jahn1, Bruno Tremblay2, Lawrence A. Mysak3
1Department of Atmospheric and Oceanic Sciences, McGill University, Burnside Hall Room 945, 805 Sherbrooke Street West, Montreal, QC, H3A 2K6, Canada, Phone 514-398-7448, Fax 514-398-6115, alexandra.jahn [at] mail.mcgill.ca
2Department of Atmospheric and Oceanic Sciences, McGill University, Burnside Hall Room 945, 805 Sherbrooke Street West, Montreal, QC, H3A 2K6, Canada, Phone 514-398-4369, Fax 514-398-6115, bruno.tremblay [at] mcgill.ca
3Department of Atmospheric and Oceanic Sciences, McGill University, Burnside Hall Room 945, 805 Sherbrooke Street West, Montreal, QC, H3A 2K6, Canada, Phone 514-398-3768, Fax 514-398-6115, lawrence.mysak [at] mcgill.ca
The Arctic Ocean has a relatively low salinity compared to other oceans, due to the large amount of runoff it receives. Hence, the export of large quantities of fresh arctic surface water and sea-ice are a source of freshwater (water with a salinity of less than 34.8) for the northern North Atlantic. Because the thermal expansion coefficient for sea water at low temperatures is very small, salinity plays a more important role than temperature for the stratification of the water column in the high latitude oceans. As a consequence, large variations in the freshwater export from the Arctic Ocean can lead to decreased deep water formation and a weaker meridional overturning circulation. As changes in the meridional overturning circulation can affect the climate world-wide, a better understanding of the mechanisms which cause these variations in freshwater export is important.
We present a detailed characterization of the freshwater budget of the Arctic Ocean and exchanges with the northern North Atlantic, as simulated by a high resolution version of the University of Victoria Earth System Climate model (UVic-ESCM). This analysis includes a 50-year average present-day freshwater budget for the Arctic Ocean as well as a thorough analysis of the interannual variability of the different terms of the Arctic Ocean freshwater balance (i.e., runoff, net precipitation, Bering Strait inflow, sea ice export, and liquid freshwater export) over the period 1950-2005. We show how this variability is related to atmospheric modes, and how atmospheric modes influence different components of the freshwater balance in the Arctic Ocean.
Arctic Research Mapping APplication (ARMAP)
George W Johnson1, Joaquin A. Aguilar2, Allison G. Gaylord3, K. Martinez4, Raed Aldouri5, Mike Dover6, Diana Garcia-Lavigne7, William F. Manley8, Robbie Score9, Craig E. Tweedie10
1Department of Biological Sciences, University of Texas at El Paso (UTEP), Systems Ecology Lab: B-325, 500 West University , El Paso, TX, 79968, USA, Phone 915-747-8816, dagenic [at] hotmail.com
2Department of Biological Sciences, The University of Texas at El Paso (UTEP), 500 West University, El Paso, TX, 79968, USA, Phone 915-747-8816, jaaguila2 [at] utep.edu
3Nuna Technologies, PO Box 1483, Homer, AK, 99603, USA, Phone 907-235-3476, nunatech [at] usa.net
4TX, USA
5Regional Geospatial Service Center, University of Texas at El Paso (UTEP), 500 West University, El Paso, TX, 79968, USA, Phone 915-747-6069, raeda [at] utep.edu
6VECO Polar Resources, 6399 South Fiddlers Green Circle, Suite 500, Greenwood Village, CO, 80111, USA, Phone 303-984-1450, Fax 303-984-1445, mike.dover [at] veco.com
7VECO Polar Resources, 8110 Shaffer Parkway #150, Littleton, CO, 80127, USA, Phone 303-984-1450, Fax 303-984-1445, diana [at] polarfield.com
8Institute of Arctic and Alpine Research (INSTAAR), University of Colorado Boulder, University Campus Box 450, Boulder, CO, 80309-0450, USA, Phone 303-735-1300, Fax 303-492-6388, william.manley [at] colorado.edu
9VECO Polar Resources, 8110 Shaffer Parkway #150, Littleton, CO, 80127, USA, Phone 303-906-0093, Fax 303-984-1445, robbie [at] polarfield.com
10Department of Biology, The University of Texas at El Paso (UTEP), 500 West University Avenue, Biology Building Room 226, El Paso, TX, 79968-0513, USA, Phone 915-747-8448, Fax 915-747-5808, ctweedie [at] utep.edu
The Arctic Research Mapping APplication (ARMAP) is an interactive, online mapping program for field-based scientific research in the Arctic. The gateway website can be found at: http://armap.org. Users can navigate to areas of interest and explore research projects by location, year, funding program, investigator, discipline, keywords, and other variables. ARMAP is dynamically linked to the Arctic Research Logistics Support Service (ARLSS) database from VECO Polar Resources, which contains over 5000 research sites, is regularly updated, and is expanding to include projects funded by a variety of agencies. Project information is displayed within the mapping application itself, with links to web pages for additional details. Users can also view: a variety of base maps (satellite imagery, topography, bathymetry, and shaded relief); framework layers (cities, roads, lakes, placename gazetteer, etc.); and scientific data (sea-ice extent, treeline, arctic vegetation, etc.). The 2D mapping application is capable of complex search queries to combine parameters. Users can copy selected data, print or export maps for presentations or publications, and choose from a "map gallery" of predefined images of interest. An online help system, a narrated tour, and FGDC metadata are provided. ARMAP will continue to improve and evolve; the 2D interface is being refined, the adoption of "best practices" will make the data widely available through additional web services, and users will soon be able to navigate in three dimensions (using Google Earth as well as ArcGIS Explorer).
With special emphasis on the International Polar Year (IPY), this service is targeted to science planners, research scientists, educators, and the general public. In sum, ARMAP goes beyond map display to analysis, synthesis, and coordination of arctic research.
Late-glacial and Holocene Biogeochemical Response to Hydrologic Changes in an Alaskan Peatland
Miriam Jones1, Dorothy M. Peteet2, Raymond Sambrotto3, Tom Guilderson4, Dorothy Kurdyla5
1Department of Earth and Environmental Sciences, Lamont-Doherty Earth Observatory of Columbia University, 204 New Core Lab, 61 Route 9W, Palisades, NY, 10027, USA, Phone 845-365-8709, Fax 845-365-8154, mjones [at] ldeo.columbia.edu
2Lamont-Doherty Earth Observatory of Columbia University, 204 New Core Lab, 61 Route 9W, Palisades, NY, 10964, USA, Phone 914-365-8420, Fax 914-365-8154, mjones [at] ldeo.columbia.edu
3Lamont-Doherty Earth Observatory of Columbia University, 61 Route 9W, Palisades, NY, 10964, USA, Phone 845-365-8402, Fax 845-365-8150, sambrott [at] ldeo.columbia.edu
4Lawrence Livermore National Laboratory, 7000 East Avenue, Livermore, CA, 94550, USA
5Lawrence Livermore National Laboratory, 7000 East Avenue, Livermore, CA, 94550, USA
Boreal peatlands have been shown to store large quantities of carbon, but climate change is threatening carbon uptake by altering atmospheric temperatures, peatland hydrology, and biogeochemical cycles. Climate change influences vegetation patterns, as plant species have different temperature and moisture thresholds. Certain plant types can also influence carbon and nutrient cycling, depending on substrate quality, oxidation availability, temperature, moisture, pH, palatability, and regrowth potential. Stable carbon and nitrogen isotopic signals preserved in peat cores have the potential to provide insight on the global carbon and nitrogen cycles, and how these have varied through time. A late-glacial and Holocene stable isotope record of carbon and nitrogen isotopes from a peatland on the Kenai Peninsula, AK was taken to document shifts in nutrients and moisture availability. Paired with a detailed record of bryophyte species change through time, estimates of pH, methane flux, and mineral availability were made. Both the carbon and nitrogen isotopes initially are depleted in the late-glacial and early Holocene, when brown mosses dominated the fen and the water table was higher. A shift to more atmospheric nitrogen isotopic values (~0%) and less negative carbon isotopic values (~5% shift) occurred when the fen became Sphagnum-dominated at ~8,800 cal BP. This shift reflects a change in nitrogen sources from combined pools to atmospheric nitrogen. The shift in moss species reflects a change in dominant water source. Early Holocene rich fen conditions prevailed when the fen was fed primarily by groundwater. Sphagnum build up above the water table caused a change in dominant water source location from groundwater to atmospheric, decreased the pH, induced a change in dominant vascular plant types, and altered biogeochemistry.
Polar Bears on Bering Sea Islands
David R. Klein1
1Institute of Arctic Biology, University of Alaska Fairbanks, PO Box 757020, Fairbanks, AK, 99775, USA, Phone 907-474-6674, ffdrk [at] uaf.edu
Explorers first visiting the St. Matthew Island group in the northern Bering Sea reported seeing numerous polar bears present there during summer when sea ice is not present in the Bering Sea. H.W.Elliott, a government scientist based at the Pribilof Islands, visited St. Matthew and Hall islands in 1874 and estimated 250 to 300 polar bears present there, including young animals. Elliot reported that many of the bears were observed to be feeding on vegetation as is common for brown bears in early summer throughout mainland Alaska. During Russian ownership of Alaska, parties of Russians were occasionally put ashore to hunt the bears for their skins. During the intensive whaling of the latter half of the 19th Century, whaling ships on their way to and from the whaling grounds are known to have stopped at St. Matthew and Hall islands to hunt walrus and polar bears. When the Harriman Expedition visited these islands in 1899, no polar bears were found. These polar bears, the only resident population present in the United States, had apparently been hunted to extinction. Fossil polar bear bones found on the Pribilof Islands indicate that there may also have been a population of polar bears resident there about 4000 years ago (Yesner et al. 2005). Current research at the University of Alaska Fairbanks is seeking answers to the following questions relating to the history, ecology, and genetic relationships of the St. Matthew Island polar bears:
1) Was plant material important nutritionally in the diet of the polar bears?
2) Were these bears genetically similar to modern polar bears?
3) Were whalers responsible for the demise of the St. Matthew bears?
4) What was the paleohistory of the St. Matthew and Pribilof Island polar bears?
5) Is reestablishment of a resident population of polar bears on St. Matthew and Hall islands a feasible conservation strategy?
St. Matthew and Hall islands are uninhabited and protected as part of the Alaska Maritime National Wildlife Refuge.
Modeling Soil Moisture Distribution in Northern Alaska: Today �100 yrs
Anna K Liljedahl1
1International Arctic Research Center, University of Alaska Fairbanks, PO Box 753851, Fairbanks, AK, 99775, USA, Phone 907-474-1951, ftakl [at] uaf.edu
A warming of the Arctic region, with thawing of carbon rich soils, will have global implications. By exerting a major control on the form of carbon (CO2 or CH4) released to the atmosphere, the soil's moisture condition will affect the strength of a positive climate feedback. At the present, annual precipitation is about the same as a desert. However, the amount of available energy limits evaporation and the annual water storage capacity in the soil, maintaining moist soil conditions throughout the summer. A climate warming can result in a drastically altered ecosystem through additional supply of energy into the system. How will the near-surface soil moisture respond to warmer soils, and a longer and/or warmer thaw season? The question requires spatially distributed, high-resolution modeling, integrating processes occurring in the physical and biological systems. TopoFlow, a physically-based hydrological model, will serve as the major tool to simulate past and future soil moisture distribution in a watershed at the northern Alaskan coast. The complex biosphere-atmosphere interactions will be evaluated by examining the inter-dependence between climate, ecosystem, hydrology, snow distribution, and active layer models. The research project is a collaborative effort under the NSF-funded project Study of Northern Alaska Coastal System (SNACS).
Carbon Flux from Melted Permafrost Cores
Lorene Lynn1
1School of Natural Resources and Agricultural Sciences, University of Alaska Fairbanks, Palmer Research Center, 533 East Fireweed Avenue, Palmer, AK, 99645, USA, Phone 907-746-1190, fslal2 [at] uaf.edu
A decrease in extent and duration of the polar ice cap coupled with increased storm intensity is causing and causing a decline in protection to the vulnerable Beaufort Sea Coast, especially in the spring and fall when the ice barrier is no longer present. The Beaufort Sea coast is eroding at a rate of one to six meters per year, washing massive quantities of carbon-rich peat soils into the sea. After a section of coastline erodes, the newly exposed ice wedges melt and the soil drains, lowering the water table and increasing the depth of the active layer. Carbon rich peat soils extend deep in to the permafrost. As these soils melt, the organic material becomes available to decomposers which can metabolize the carbon into methane and carbon dioxide. In order to understand the fate of the stored organic carbon, permafrost cores were collected at three sites: Barrow, Prudhoe Bay, and Barter Island. The cores were melted over a 24 hour period and released methane and carbon dioxide were measured. At maximum values, carbon dioxide was 100 times greater than methane. Melt water from the cores was tested for dissolved organic carbon (DOC) concentrations. Values for DOC ranged from 0.1 - 450.0 mg/L; the range being highly dependent on the total ice content present in each sample. Total carbon was also measured in order to calculate the complete carbon balance in the permafrost cores. In order to better understand what portion of the permafrost cores exists as ice and will drain from the soil when melted, soil moisture was calculated. This research project is a collaborative effort under the NSF-funded project Flux and Transformation of Organic Carbon across the Eroding Coastline of Northern Alaska.
International Polar Year Data and Information Services
Mark S. McCaffrey1, Mark A. Parsons2
1CIRES, University of Colorado at Boulder , UCB 449, Boulder, CO, 80309, USA, Phone 303-735-3155, Fax 303-735-3644, mark.mccaffrey [at] colorado.edu
2National Snow and Ice Data Center (NSIDC), University of Colorado Boulder, University Campus Box 449, Boulder, CO, 80309, USA, Phone 303-492-2359, Fax 303-492-2468, parsonsm [at] nsidc.org
The International Polar Year Data and Information Service (IPYDIS) is an international federation of data centers, archives, and networks working to ensure proper stewardship of IPY and related data. The National Snow and Ice Data Center seeks to act as a coordination office for the IPYDIS to ensure the long term preservation of and broad, interdisciplinary, and non-expert access to IPY data. The IPYDIS is guided by the IPY Data Policy and Management Subcommittee, which is presently developing an overall IPY data strategy. The IPYDIS, through its Data Coordination Office, plans to visibly track the data flow for IPY. In collaboration with the International Polar Year Programme office, it will develop a data registry that will continue throughout the IPY. Ultimately, this site may provide a portal to IPY and related data. In addition, the IPYDIS will coordinate the development of a template for narrative educational metadata, or Data Stories, with IPY project leads in support of goals of the IPY Education, Outreach and Communications (EOC) Subcommittee, which seeks to demystify how data are collected and research is conducted. The initial Data Stories will focus on sea ice research and data.
Facilitating Collaborative Scientific and Technical Research in the Arctic Sciences and Geosciences
Laura M. Meany1, Erik A. Edlund2
1Cooperative Grants Program, U.S. Civilian Research & Development Foundation, 1530 Wilson Blvd., Suite 300, Arlington, VA, 22209, USA, Phone 703-526-2324, Fax 703-526-9721, Lmeany [at] crdf.org
2Cooperative Grants Program, U.S. Civilian Research & Development Foundation, 1530 Wilson Blvd., Suite 300, Arlington, VA, 22209, USA, Phone 703-600-3440, Fax 703-526-9721, Eedlund [at] crdf.org
The U.S. Civilian Research and Development Foundation (CRDF) is a private, nonprofit, grant-making organization created in 1995 by the U.S. Government through the National Science Foundation. CRDF promotes international scientific and technical collaboration, primarily between the United States and Eurasia, through grants, technical resources, and training.
The Foundation's goals are to support exceptional research, offer scientists alternatives to emigration, and strengthen the scientific and technological infrastructure in Eurasia. CRDF achieves these goals by advancing the transition of foreign weapons scientists to civilian work, moving applied research to the marketplace, and strengthening research and education in universities abroad.
CRDF offers two unique services and a program that provide support to U.S. and Russian scientists engaged in collaborative arctic and geoscience related research.
- Under a contract with the National Science Foundation, CRDF assists Office of Polar Programs (OPP) and Geosciences Directorate (GEO) grantees and collaborators by providing an office and personnel in Moscow. Using this staff and in-country resources, NSF grantees receive help in identifying and communicating with individual and institutional partners, navigating government agencies, facilitating travel and visas, and providing on-site office support to visiting U.S. travelers.
- CRDF's GAP Services enable U.S. government agencies, universities, and other organizations to utilize CRDF's financial and administrative infrastructure to transfer payments, purchase and deliver equipment and supplies, and carry out other project management services to collaborators in Russia and elsewhere in Eurasia.
- The Cooperative Grants Program (CGP) allows U.S.-Russian collaborators in arctic sciences and geosciences to apply for two-year R&D grants.
Traditional Ecological Knowledge, Persistent Organic Pollutants and Its Impact on Environmental Science and Policy in Alaska
Nazune Menka1
1American Indian Student Support, Arizona State University, 2243 W. Concho Circle, Mesa, AZ, 85202, USA, Phone 808-389-0777, nazune [at] asu.edu
Traditional Ecological Knowledge (TEK) is a valid scientific tool that many academic research institutions have used to initiate scientific and academic studies. In particular the issue of climate change in the Arctic, where the State of Alaska resides, has illustrated that TEK is an essential tool and has not only been used to initiate research studies but has become "The National Threat Advisory" for climate change and the failing ecosystems of the planet. Research is showing a long list of persistent organic pollutants (POPs) that have infested the land, animals and Alaska Native communities.
These chemicals originate from industrial processes all over the world and are carried through normal air pathways up to the Arctic. They are then ingested by ocean biota, marine and land mammals and inevitably humans. POPs have a unique ability to bioaccumulate in the environment as they are highly resistant to degradation and are lipophilic, causing them to be stored in fatty tissues and therefore gives them the ability to make their way up the food chain. While there is little research as to the effects of these chemicals on human health there is information on health effects being compiled from animal species that will help us understand the possible effects on humans.
Influx of Freshwater Runoff to the Northern Atlantic Ocean from East Greenland
Sebastian H. Mernild1
1IARC and WERC, University of Alaska Fairbanks, PO Box 750292, Fairbanks, AK, 99775, USA, Phone 907-474-2787, Fax 907-474-7041, fxsm [at] uaf.edu
The arctic climate is changing. Hydrological processes are changing and evolving in response to these changing conditions. In the Arctic the rough terrain and harsh climatic conditions yield a lack of cryospheric and hydrological knowledge. The limited data of such key components is a serious impediment to the hydrological research at East Greenland e.g., the amount and variation in cryosphere storage change and in influx of freshwater runoff to the North Atlantic Ocean. In arctic Greenland, the terrestrial cryosphere storage (snow, ice, and permafrost) does have an important influence in the high-latitude freshwater runoff contribution to the ocean.
Analyses of freshwater runoff from the only two East Greenland catchments have been done by glacier and snow observations, water flow observations, and experiments through the glaciers and the landscape, indicating glacier recession both places. Total freshwater runoff from the southern catchment (the Mittivakkat catchment) was ~3.7*10-2 km3 y-1 and from the northern catchment (the Zackenberg catchment) ~21.9*10-2 km3 y-1, high dominated by the percentage of glacier cover. The glacier recession dominates the mean annual catchment runoff by 30 to 90%. The total freshwater input up-scaled to the North Atlantic Ocean is ~450 km3 y-1 (1999-2004). The future (2071-2100) climate impact assessment based on the IPCC A2 and B2 scenarios modeled in HIRHAM indicates an increasing mean annual East Greenland air temperature by 2.7 degrees C. The 2071-2100 mean annual freshwater input to the North Atlantic Ocean is assessed to ~670 km3 y-1, an increase by approximately 50% from today's values, highly based on changes in air temperature rather than in precipitation and evapotranspiration.
Arctic Science Discoveries
National Science Foundation1
1Office of Polar Programs, 4201 Wilson Boulevard, Arlington, VA, 22230, USA, http://www.nsf.gov/od/opp/
Our understanding of the Arctic has increased enormously over the past five decades of intense research, but much remains to be learned, and new discoveries await researchers who study this unique region. The Arctic Sciences Section of the National Science Foundation funds basic research of the Arctic through the Arctic Natural Sciences, Arctic Social Sciences, and Arctic System Science programs, with field research support from the Research Support and Logistics program. Some important research results are presented both as answers to important questions and leads to future research directions:
- Studying Arctic Change: The Study of Environmental Arctic Change(SEARCH) is an interagency, interdisciplinary, multiscale program to study changes occurring in the Arctic and their potential impacts.
- A look at Ringed Seal Migration: Working with Alaska Native hunters, researchers captured a ringed seal and attached a satellitetracking device to get the first-ever look at spring migration in this species as it moves northward with the melting ice of the Chukchi Sea.
- Photochemistry in Greenland Snow: Scientists have discovered that light-mediated chemical reactions (photochemistry) occur at the air-snow interface and significantly impact the chemical composition of air trapped in ice and of the air overlying the snow.
- Small Streams on the Move: Researchers have discovered that small streams contribute more to removing nutrients such as nitrogen from water than do their larger counterparts. The findings are based on data collected initially from streams in NSF's Arctic Tundra Long-Term Ecological Research site in Alaska and subsequently from 12 sites across the country.
- Living Conditions in the Arctic: This is an international effort involving a partnership of researchers and indigenous organizations across the Arctic. The purpose is to advance our understanding of changing living conditions among Inuit and Saami peoples and the indigenous peoples of Chukotka.
- Life on the Gakkel Ridge: The Gakkel Ridge is the slowest spreading center in the world, giving scientists the opportunity to explore the earth's inner layers as the mantle spreads at about 1cm per year onto the ocean floor near the North Pole.
- Understanding the Arctic Ocean: The Western Arctic Shelf BasinInteractions(SBI) project is investigating the impact of global change on physical, biological, and geochemical processes over the Chukchi and Beaufort Sea shelf basin in the western Arctic Ocean. Closely affiliated is the Chukchi Borderlands project to study the region where relatively cold, fresh, and nutrient-rich water from the Pacific Ocean meets warmer, saltier and deeper water from the Atlantic Ocean over a bottom tortuously rife with slopes, ridges and deep-sea plateaus.
The International Polar Year
National Science Foundation1
1Office of Polar Programs, 4201 Wilson Boulevard, Arlington, VA, 22230, USA, http://www.nsf.gov/od/opp/
The International Polar Year 2007-2008 is envisioned to be an intense, coordinated field campaign of polar observations, research, and analysis that will be multidisciplinary in scope and international in participation. IPY 2007-2008 will provide framework and impetus to undertake projects that normally could not be achieved by any single nation. The National Science Foundation (NSF) was designated by the President's Office of Science and Technology to be the lead U.S. agency in organizing IPY activities.
The Circumpolar Active Layer Monitoring (CALM) Network
Frederick E. Nelson1, Nikolay I. Shiklomanov2, Kenneth M. Hinkel3, Jerry Brown4, Galina Mazhitova5
1Department of Geography, University of Delaware, 207 Pearson Hall, University of Delaware, Newark, DE, 19716, USA, Phone 302-831-0852, Fax 302-831-6654, fnelson [at] udel.edu
2Department of Geography, University of Delaware, 216 Pearson Hall, Newark, DE, 19716, USA, Phone 302-831-1314, Fax 302-831-6654, shiklom [at] udel.edu
3Department of Geography, University of Cincinnati, 400 F Braunstein Hall, PO Box 210131, Cincinnati, OH, 45221-0131, USA, Phone 513-556-3430, Fax 513-556-3370, kenneth.hinkel [at] uc.edu
4International Permafrost Association, International Permafrost Association, PO Box 7, Woods Hole, MA, 02543-0007, USA, Phone 508-457-4982, Fax 508-457-4982, jerrybrown [at] igc.org
5Insitutute of Biology, Komi Science Center, Russian Academy of Sciences, Kommunisticheskaya St 28, Syktyvkar, -, 167982, Russia, Phone +7 8212 245115, Fax +7 8212 240163, galina_m [at] ib.komisc.ru
The Circumpolar Active Layer Monitoring (CALM) program is one of several global-change programs affiliated with the International Permafrost Association (IPA). CALM was initiated in the early 1990s to track possible changes and trends in the seasonally frozen ("active") layer in the permafrost regions. Widespread, large-magnitude increases in the thickness of the active layer induced by climatic warming could liberate carbon sequestered in near-surface permafrost, create irregular topography ("thermokarst terrain") in areas of ice-rich permafrost, damage human infrastructure on the surface, and induce pronounced ecological changes.
CALM is a hypothesis-driven program that monitors active-layer thickness and shallow ground temperature, coordinates field experiments, and provides data for use by investigators involved in a wide-range of cold-environment research and modeling activities. The CALM network is currently comprised of more than 150 sites distributed throughout the Arctic, parts of Antarctica, and several mountain ranges of the midlatitudes. Efforts to expand the number and capabilities of sites in the Southern Hemisphere (CALM-S) are underway. Instrumentation and data-acquisition methods include monitoring the soil thermal and moisture regimes with automatic data loggers, mechanical probing of the seasonally thawed layer at specified spatial and temporal intervals, frost/thaw tubes, and a variety of instruments for measuring frost heave and thaw subsidence. Several groups of sites have been used to create maps of active-layer thickness, and estimates of the volume of thawed soil at regional scales. The CALM network has also provided a large amount of data pertaining to cryostratigraphy, cryoturbation, and soil carbon. Data obtained from the network have been used in validation procedures for hydrological, ecological, and climatic models, at a variety of geographic scales. Data are archived at the Frozen Ground Data Center (http://nsidc.org/fgdc/) in Boulder, Colorado.
CALM is sponsored by the U.S. National Science Foundation's Office of Polar Programs. CALM is linked with many other global-change programs through the network of observatories known collectively as the Global Terrestrial Network for Permafrost (GTN-P), a network under the WMO Global Climate Observing Network (GCOS). With its sister programs Thermal State of Permafrost (TSP), Antarctic Permafrost, Periglacial, and Soil Environments (ANTPAS), Carbon Pools in Permafrost Regions (CAPP), and Arctic Coastal Dynamics (ACD), CALM forms a comprehensive effort on the part of the International Permafrost Association to monitor, understand, and predict the effects of environmental change in the world's permafrost regions. CALM is a major component of the IPA's coordinated program for the International Polar Year. Detailed information about the CALM program can be found at http://www.udel.edu/Geography/calm/.
Hunting Bowhead in Northern Alaska 1980-2005: Inupiat Adaptation and Resilience to Climatic Variability
Craig Nicolson1, Stephen Braund2, Craig George3, Jack Kruse4, Sue Moore5, Carin Ashjian6
1Department of Natural Resources Conservation, University of Massachusetts Amherst, 160 Holdsworth Way, Amherst, MA, 01003, USA, Phone 413 545-3154, Fax 413 545-4358, craign [at] forwild.umass.edu
2Stephen Braund and Associates, PO Box 101480, Anchorage, AK, 99510-1480, USA, Phone 907-276-8222, Fax 907-276-6117, srba [at] alaska.net
3Department of Wildlife Management, North Slope Borough, PO Box 69, Barrow, AK, 99723, USA, Phone 907-852-0350, Fax 907-852-9848, cgeorge [at] co.north-slope.ak.us
4Institute of Social and Economic Research (ISER), University of Alaska Anchorage, 117 North Leverett Road, Leverett, MA, 01054, USA, Phone 413-367-2240, Fax 413-367-0092, afjak [at] uaa.alaska.edu
5National Marine Mammal Laboratory, National Oceanic & Atmospheric Administration (NOAA), 7600 Sand Point Way NE, Seattle, WA, 98115, USA, Phone 206-526-4047, Fax 206-526-6615, sue.moore [at] noaa.gov
6Department of Biology, Woods Hole Oceanographic Institution, Redfield 2-46, MS #33, 266 Woods Hole Road, Woods Hole, MA, 02543, USA, Phone 508-289-3457, Fax 508-457-2134, cashjian [at] whoi.edu
Bowhead whales are a vitally important cultural and subsistence resource in the Alaskan communities of Barrow and Kaktovik. In the light of current projections of a warming arctic, we have been examining how whale migration, regional climate change, variability in local weather conditions and policy are likely to affect the success of whale hunting in these two communities. Although in the central Beaufort Sea the offshore distance of the whales' migration path is influenced by summer sea ice extent, at Barrow and Kaktovik whales are close to shore irrespective of the regional sea-ice extent. We found that in the spring whale hunting season at Barrow (April 20-May 31), wind speed and direction both have an important influence on hunting success. However, in fall the data indicate a) that wind direction is not strongly linked to hunting success, and b) even though at a daily scale the average wind speed does influence the probability that hunters will land a whale, the overall success of the Barrow fall hunting season (as measured in total number of whales landed) during 1980-2005 was much more strongly affected by International Whaling Commission policy (especially from 1980-1992), by the community's need for whales after the Spring hunt, and by the timing of the hunt than by wind speed. Finally, we show that whale hunting is a strongly adaptive system, responding to feedbacks from science and from hunters' experience in previous years. We conclude that whale hunting in Barrow and Kaktovik seems remarkably resilient to climate variability and change.
The Narwhal Tusk, Potential as an Arctic Water Probe and Weather Station: Collaborative and Integrative Studies of Science and Inuit Traditional Knowledge
Martin T. Nweeia1
1Restorative Dentistry and Biomaterials Sciences, Harvard School of Dental Medicine, Marine Mammal Program Smithsonian Institution, PO Box 35, 6 New Street, Sharon, CT, 06069, USA, Phone 860-364-0200 , Fax 860-364-5606, martin_nweeia [at] hsdm.harvard.edu
Biological and behavioral studies of the narwhal may contribute valuable insights and information to the understanding of a changing arctic environment. Broad based collaborations with the integration of myriad disciplines has enabled this work to comment on osteocranial structures, and teeth as well as narwhal behavioral modifications in response to ocean and climate change.
Studies of narwhal tusk microanatomy reveal structural components with hydrodynamic sensory capabilities. A network of microtubules connects the inner nerve and blood supply of this tooth to its external environment. Collaborating scientists at the Paffenbarger Research Center (PRC) at the National Institute of Standards and Technology examined two freshly harvested tusks and discovered millions of such connective, fluid filled pathways that are capable of detecting changes in water and air temperature, pressure and osmotic gradients. The tusk surface is also capable of sensing tactile stimuli. Using the theorized model of tubule fluid detection discovered by Swedish scientist Martin Brannstrom, narwhal are capable of detecting changes in its water and air environments that most mammals can only sense in pathologic conditions. PRC investigators also examined position resolved sections of narwhal tusk by Fournier transform infrared fluorescence microspectroscopy which revealed an opposite structural modeling to normal mammalian teeth. Narwhal tusks have lower mineral to collagen (m/c) ratios in their outer dentin circumference and the inner core has a high m/c ratio giving it a rigid core surrounded by a flexible outer covering. Analysis of tissue hardness and flexibility reveal bending properties that enable an eight foot tusk to flex approximately one foot in all directions.
These microanatomical tusk characteristics generate thought about function as it relates to evolutionary adaptation. The existence of dentin dates to the late Cambrian period, approximately 510 million years ago, at that time expressing a biodiversity that greatly exceeded the subsequent 450 million years and present day variation. Ordovician jawless fish had exoskeletons derived from dentin material that had the ability to detect temperature, osmotic gradients and tactile sensation. Though the teeth of mammals have this tissue and the associated capability for sensory perception, the narwhal exhibits an unusual expression and configuration of these tubules.
Embryologic studies, cited in the literature, describe tooth buds for six pairs of teeth, four that are somehow switched off genetically and one that is minimal in adult development. Despite a diet of larger fish like Greenland halibut and Arctic cod, the narwhal has no teeth erupted in its mouth for chewing. Its adaptation of turning on a single growth center producing such a disproportionate size tusk with no intraoral function, and having such an unusual microanatomy and expression, is explored in this investigation of narwhal tusk function.
Collection of Inuit Traditional Knowledge, from ten communities in Northeastern Baffin Island and Northwestern Greenland that have a strong association with narwhal, parallels the scientific investigations. Traditional Knowledge has guided, confirmed and challenged the findings of this study in many and varied disciplines of scientific pursuit. Inuit descriptions of anatomic variation, observations of behavior, and the effects of migration patterns and timing are noteworthy in the discussion of tusk function and narwhal behavior, and add to the overall knowledge and understanding for this arctic marine mammal. Findings from this investigation demonstrate the value of integrating science and social science, and combining the analytic mind of a trained scientist with the perception, observation and experience of Inuit on the land.
Narratives of Environmental Change from Wetland, River and Lake Communities in Iceland and Norway
Astrid E. Ogilvie1, Oyvind Nordli2, Gisli Palsson3, Thomas H. McGovern4, Arni Einarsson5, Orri Vesteinsson6, Trausti Jonsson7, Ian A. Simpson8, Jennifer Brown9
1Institute of Arctic and Alpine Research, University of Colorado, Campus Box 450, 1560 30th Street, Boulder, CO, 80309-0450, USA, Phone 303-492-6072, Fax 303-492-6388, Astrid.Ogilvie [at] colorado.edu
2Norwegian Meteorological Institute, Box 43, Blindern, Oslo, N-0313, Norway, oyvind.nordli [at] met.no
3Department of Anthropology, University of Iceland, Oddi, Reykjavik, IS-101, Iceland, Phone +354-5254253, Fax +354-5254253, gpals [at] hi.is
4Department of Anthroplogy, Hunter College, CUNY, 695 Park Avenue, New York, NY, 10021, USA, Phone 212-772-5654, Fax 212-772-5423, nabo [at] voicenet.com
5Myvatn Research Station, Myvatn, IS-660, Iceland, arnie [at] hi.is
6Institute of Archaeology, University of Iceland, Barugotu 3, Reykjavik, IS-101, Iceland, Phone +354-551-1033, Fax +354-551-1047, orri [at] instarch.is
7Department of Research Processing, The Icelandic Meteorological Office, Bustadavegi 9, Reykjavik, IS-150, Iceland, Phone +354-522-6000, Fax +354-522-6002, trausti [at] vedur.is
8School of Biological and Environmental Sciences, University of Stirling, University of Stirling, Stirling, FK9 4LA, UK, i.a.simpson [at] stir.ac.uk
9School of Biological and Environmental Sciences, University of Stirling, University of Stirling, Stirling, FK9 4LA, UK, jennifer.brown [at] stir.ac.uk
Records of increasing temperatures, melting glaciers, reductions in extent and thickness of sea ice, thawing permafrost, and rising sea level all highlight the recent warming in the Arctic and Subarctic. Evidence for these changes has now become overwhelming (as documented in the recent IPCC Working Group I report). The changes are not limited to Polar ocean and coastal regions, but also affect other areas currently covered by ice and snow. Thus, for example, rivers fed by mountain glaciers could run dry or change their course. Social change is intertwined with environmental change and human and animal populations are impacted across the Arctic and Subarctic. This poster highlights work drawn from several research projects which have an international multidisciplinary approach to understanding environmental change in wetland, river and lake communities in Iceland and Norway. These projects cover many scientific disciplines including historical climatology, meteorology, archaeology, anthropology, biology, ecology, soil science and tephrochronology. Information is also provided by traditional ecological knowledge. Areas of focus are the Myvatn wetland area in Iceland and lake/wetland communities in the Oppland district of Norway.
Myvatnssveit (the "Myvatn district") lies on the western border of the volcanic zone which cuts across northeastern Iceland from north to south, and which is an extension of the Mid Atlantic Ridge. The district takes its name from the lake Myvatn (literally "midge water"). Myvatn is a large shallow lake of about 37 sq km with about 50 islands and islets in the lake. The surrounding landscape is shaped by volcanism, and lake Myvatn itself was created c. 2000 years ago by a volcanic eruption which poured large volumes of lava over the district. The lake and its outflowing river, the Laxá, are renowned as a breeding and moulting ground for a large number of species of duck and other water birds. Lake Myvatn and the Laxá river were protected by law in 1974, and in 1978 placed on the RAMSAR list of wetlands of international importance (http://www.ramsar.org/). The area seems to have been one of the first to be settled in Iceland's landnám or early settlement period. The district is relatively isolated. In 1984, the population numbered 590, but by 1996 it had dropped to 470. Approximately 200 people live in the village of Reykjahlí∂. Until the early part of the twentieth century, the inhabitants lived almost entirely on the proceeds of the land by farming and fishing for trout (Myvatnsilungur) in Lake Myvatn. However life has changed radically in recent times. Because of its volcanic nature, Iceland is well suited to the use of geothermal energy and geothermal power plants have been established at Krafla and Bjarnarflagi. The Krafla station in particular was a topic of debate from the outset and its construction provoked a major public controversy. The plant started operations early in 1977. Landsvirkjun, the Icelandic Power Company, bought the plant from the Icelandic state in 1985. These plants provide employment for about 20 people. Very few plants use fossil fuels such as coal or oil as their energy source. In this way, Iceland obtains almost its entire power supply from clean energy resources. Not surprisingly, there has been concern about the possible effect of increased geothermal development and effluents on the natural environment in and around Lake Myvatn only 2 km away and potential effects are monitored carefully (see e.g. Thorbergsdóttir, et al., 2004). With Myvatn's scenically beautiful and geologically interesting landscapes, coupled with the outstanding bird life, it is not surprising that tourism is a steadily growing business. There are several hotels and guest houses as well as restaurants, camping sites and other small companies connected to tourism. This area has been chosen for study because the changes occurring there are currently rapid and far-reaching - agricultural practices that have changed little from settlement times are in sharp contrast to the recently established generation of geothermal power.
The district of Oppland (meaning "Highland") in southeastern Norway stretches from broad farmlands in the south to the Rondane, Dovre and Jotunheimen mountain ranges in the north. It is one of only two districts in Norway that do not have a sea coastline. The total population of Oppland is 184,000 (compare with Iceland's total population of approximately 300,000). The area includes high mountain terrain as well as tracts of forests and also agricultural landscapes with a focus on sheep and cattle as the main farming activity. It has some 48 lakes of which one, Randsfjord, will receive special attention here (Nordli et al., 2007). The area has a rich cultural heritage, and archaeological findings show a large and well-organized community in existence 3000 years ago. It thus forms a long-established farming community where many traditional practices are maintained. Thus, for example, seterbruk (transhumance) still exists, that is, bringing livestock up to the lush mountain pastures for the summer months. Indeed, it is the Oppland region of Norway where transhumance is most common. However, this practice is rapidly dying out. About one-third of the populace still derive their livelihood from farming, primarily meat and milk production. Other important economic practices are forestry, the tourist industry and the production of rakfisk, preserved trout. Fish in lakes and streams provided an important supplement to the diet in the past, and continue to do so today. Trout and perch are the main species found. Another fish, the so-called "Common whitefish" (Coregonus lavaretus, Norwegian name Sik) found in certain waters in the region is on the World Conservation Union's list of threatened species. Fishing is also a recreational activity and is part of the attraction of the area for tourists. As in Iceland, tourism is a becoming a booming industry, and is taking the place of many traditional economic practices. Also, as in Iceland, the use of hydroelectric power is being developed fast in the larger watercourses of Oppland.
Information regarding environmental and social change for the Oppland and Myvatn areas will be highlighted here. Data sources include documentary historical evidence such as weather diaries and letters, as well as environmental and climate data from systematic modern observations. Other sources of information range from zooarchaeological data in the form of well-dated animal bone collections, to present-day agronomic, socioeconomic, and population data, as well as local knowledge from key informants.
References
Nordli, Ø. Lundstad, E, and Ogilvie, A.E.J. 2007. Late Winter-Early Spring Temperature Reconstructions for Southeastern Norway based on Lake Ice Break-Up. Annals of Glaciology 46. In Press.
Thorbergsdóttir, I.M., Reynir Gíslason, S., Ingvason, H.R. and Einarsson, Á. 2004. Benthic oxygen flux in the highly productive subarctic Lake Myvatn, Iceland: In situ benthic flux chamber study. Aquatic Ecology 38, 177-189.
Video Podcast Project at Kate Waller Barrett Elementary in Arlington, Virginia
Gabby Pena1, Alexis Reyes2, Laurie Sullivan3
14th Grade, Kate Waller Barrett Elementary School, 4401 North Henderson Road, Arlington, VA, 22203, USA, Phone 703-228-8547, Fax 703-351-0023
24th Grade, Kate Waller Barrett Elementary School, 4401 North Henderson Road, Arlington, VA, 22203, USA, Phone 703-228-8547, Fax 703-351-0023
3Project Discovery Teacher, Kate Waller Barrett Elementary School, 4401 North Henderson Road, Arlington, VA, 22203, USA, Phone 703-228-8547, Fax 703-351-0023
Teachers: Laurie Sullivan, Renee Shaw, and Angela Lambert
4th Grade Student Authors of podcasts relating to Antarctica
- Expedition to Antarctica with Mr. Miller by Gabby Pena
- Expedition to Antarctica by Alexis Reyes
The video podcasts can be viewed online at http://carpecamera.blogspot.com
National Science Foundation Einstein Fellow Allan Miller involved Barrett fourth graders in his expedition to Antarctica in December and January 2006/2007. He met with the students before the expedition and invited them to try on his cold weather gear. While in Antarctica he and the students communicated by posting messages on the PolarTREC website (http://www.polartrec.com) and by satellite phone calls. Students conducted science investigations in the Discovery Lab related to the expedition. Mr. Miller visited with the students upon his return. The students showed Mr. Miller the video podcasts they had created about Antarctica. These video podcasts were featured at the NSF Science and Engineering Open House on February 5, 2007.
The student projects may be viewed at http://carpecamera.blogspot.com
Precipitation Changes, Uncertainties, and Associated Impacts to the Arctic Environment
Michael A. Rawlins1
1Complex Systems Research Center, University of New Hampshire, Morse Hall, Durham, NH, 03824-0188, USA, Phone 603-862-4734, Fax 603-862-0188, rawlins [at] eos.sr.unh.edu
Recent assessments of the arctic environment suggest that significant changes have occurred, due primarily to an increase in air temperature almost twice the global average rate over the past century. Analysis of records from meteorological stations and from models simulations show a positive trend in annual precipitation across much of the Arctic, with the strongest increases evident over the winter months. A trend toward more extreme precipitation events is also noted in the historical station record. Uncertainties in arctic precipitation estimates are attributable to both the sparse nature of meteorological stations networks and rain-gauge undercatch of solid precipitation in windy environments.
Changes anticipated to occur over the next century follow the trajectory of past trends. Although model spread is relatively high, increases in annual precipitation of 10-30% are suggested based on the Special Report of Emissions Scenarios (SRES) A1B storyline of future greenhouse gas emissions. Projected arctic precipitation increases will likely exceed global trends due to enhanced storminess at high latitudes. This presentation describes precipitation changes that have occurred over the past century, the challenges in characterizing arctic precipitation variability and change, and projections for future trends. Changes in precipitation seasonality and the impact on river discharge and water resource availability are also explored.
Identification of Dominant Patterns in Pan Arctic River Discharge Trends
Asa K. Rennermalm1, Eric F. Wood2
1Department of Civil and Environmental Engineering, Princeton University, Engineering Quad, Princeton University, Princeton, NJ, 08544, USA, Phone 609-356-2659, arennerm [at] princeton.edu
2Department of Civil and Environmental Engineering, Princeton University, Engineering Quad, Princeton University, Princeton, NJ, 08544, USA, Phone 609-258-4675, Fax 609-258-2799, efwood [at] princeton.edu
In the 21st century, climate change due to anthropogenic release of greenhouse gases is expected to be most pronounced in the arctic region. That arctic climate change already might be underway is indicated by 20th century increase of Eurasian river discharge into the Arctic Ocean. However, there is a spatial variability in arctic discharge trends exemplified by the late 20th century decreased river discharge into Hudson Bay. The causes for the spatially varying arctic discharge trends and are largely unknown. Finding explanations for 20th century discharge trends have been difficult because of the sparse temporal and spatial coverage of arctic environmental and hydrological data.
We propose that trend attribution can be aided by the identification of regions within the arctic drainage basin that have similar trend characteristics. Here we present a framework to seek for dominant patterns in arctic river discharge trends. The framework combines a simple description of trends in station discharge measurements with a clustering and classification technique to reduce the complexity of arctic discharge data. Indeed, with this method we are able to identify dominant trend patterns of pan arctic discharge trends that also show some spatial patterns. Thus, the presented framework has the potential to significantly simplify the trend attribution process by reducing the problem from finding the cause of the trends in every single river basin to only finding the cause to a few dominant trend patterns. The limitations to trend attribution set by data gaps in time and space may be solved for by using environmental and hydrological data from all regions where a dominant trend pattern appears.
High Resolution Analysis of Laminated Lacustrine and Marine Sediments in Cape Hurd Lake, Devon Island, Nunavut, Canada: a Contribution to the ARCSS 2,000 Year Synthesis of Climate Variability from Arctic Lakes
Mike Retelle1
1Department of Geology, Bates College, Carnegie Science Center, 44 Campus Avenue, Lewiston, ME, 04240, USA, Phone 207-786-6155, Fax 207-786-8334, mretelle [at] bates.edu
A fundamental task of the NSF-ARCSS sponsored initiative "A Synthesis of the Last 2000 Years of Climatic Variability from Arctic Lakes" is to reconstruct series of high-resolution records of arctic temperature using various physical, biological and biogeochemical proxies for the past 2,000 years. In this fashion, the present warming trend and its predicted impacts (ACIA, 2004) can be assessed in the context of past cold and warm events ( i.e. the Little Ice Age and Medieval Warm Period) and a more clear understanding of temporal and spatial climatic variability in the arctic system can be attained. In the 2K initiative, 14 PI's and 11 collaborators focus their efforts on approximately 30 lake sites spanning from Beringia across the North American arctic to Svalbard. The detailed lacustrine records will provide new and detailed paleoclimate data to assist climate modeling experiments that will explore climate forcing mechanisms and controls such as volcanism, solar irradiance, and inherent modes of climate variability to explain significant temporal and spatial patterns in the proxy records.
This poster presents results of geochemical and sedimentological analysis of sediments from Cape Hurd Lake, Devon Island (74o37'N 90 15'W) in the Canadian high arctic. Results from the first phase of this study focused on the analysis of several short cores recovered in spring 2003. The short core record extends back to about ca. 600 B.P. (Putnam, 2004; Retelle, et al., 2006) and was presented at the Arctic Workshop in Boulder in 2006. In this poster, we present a composite record using detailed analyses from both the short core and the upper meter of a 6 meter-long vibracore, to extend our series to span the last two thousand years.
Cape Hurd Lake is a relatively small (~2.0 km2) density-stratified coastal isolation basin situated at sea level adjacent to Lancaster Sound, the eastern arm of the Northwest Passage on southwestern Devon Island. The basin is separated from the sea by a narrow bedrock sill mantled by shingle beach deposits that rise from west to east from sea level to approximately 70 meters asl. The basin is connected by a tidal inlet to Lancaster Sound during the summer melt season, allowing tidal circulation into the basin when sea ice melts and retreats from the channel mouth. Two inlet streams feed the lake. The main inlet on the north shore drains from a plateau ice cap while a minor snowmelt-fed inlet enters along the west shore of the lake.
The basin has evolved through the Holocene from an open glacial-marine inlet to a density-stratified isolation basin (Saenger, 2002). Today, salinity in the epilimnion varies seasonally due to input of glacial meltwater from the plateau ice cap. Prior to initiation of seasonal runoff in 2003, a cap of oxygenated saline water (32 ppt) lay over anoxic and more saline (40 ppt) bottom water which was found to a depth of 55 meters.
In spring 2003, three short cores were recovered using Glew and Aquatic Research gravity coring systems from the 50 meter deep central basin site where a 6 meter long Rossvelder vibracore had been recovered in 2001 (Saenger 2002). One short core provided thin sections and preliminary lower resolution downcore analysis of grain size, %LOI, and biogenic silica as described by Putnam (2004). One of the additional adjacent surface cores was recovered within several meters of Putnam's core and was subsampled in detail (0.5 cm interval) for the 2k project. Both surface cores contain approximately 35 cm of finely laminated clayey silt overlying a 10cm-thick massive layer. Analysis of the long core was undertaken on the uppermost meter of the vibracore and on a 1 meter u-channel sample taken from the core for paleomagnetic studies (Stoner et al., 2004).
Multiproxy analyses of the sediments include grain size, magnetic susceptibility, biogenic silica (BiSi), %LOI, %OC, C/N, 13C and 15 N of organic matter. Non-destructive high-resolution elemental analysis (10 micron stratigraphic sampling) was also undertaken on the u-channel samples from both the surface and vibra cores using an ITRAX core scanning XRF, housed in the GIRAS laboratory at the Centre Eau, Terre & Environnement, Quebec, courtesy of Dr. Pierre Francus.
Preliminary results are outlined below:
Lithostratigraphy and geochronlogical control
- The sediments in the cores are mostly finely laminated couplets of terrigenous carbonate mud and diatomaceous layers separated by occasional thicker turbidites and massive layers. Lamination counts and thickness were initially done for this core site by Putnam (2004) and recounted using various sets of criteria. A varve count of approximately 300 years for the surface core recognized multiple intra-annual pulses within varves as events within a melt season and best matches the 210Pb results.
- The age model for the core was constructed using varve count for the upper 30 cm is approximately 300 years while the lower portion of the stratigraphy below the laminated zone to 100 cm is constrained using an 14C AMS age on fish bone in sediments at 135 cm depth in the long core yielded an age of 3,310 +/- 40 (3055+11 calendar years). Several other macrofossils including plant debris and fishbone have been removed from the cores and have been submitted for AMS 14C analysis.
- Detailed XRF multi-element scanning provides a wealth of data on major cations and trace elements. Our first focus is an attempt to utilize Ca as a proxy for rock flour flux due to the predominance of limestone bedrock in the Cape Hurd watershed. In both the long and short cores Ca levels are out of phase with biogenic silica and 13C indicating the predominance of marine phytoplankton as the major sediment component, overwhelming terrestrial sediment from the ice cap during these phases.
Geochemistry:
- C/N values of the organic matter in the short core ranges between 9 and 14, representing a predominantly aquatic source (i.e., phytoplankton or sea ice algae), with periods of higher terrestrial input possibly corresponding to depths with higher C/N values. In the long core C/N shows a prominent shift at 35 cm depth from values from 8 to 10 to values averaging 12 to 13, perhaps indicative of increased terrestrial input.
- 13C values of organic matter from the short core sediments range between -28 and 24 per mil with more enriched values in the upper third of the core. In the long core, the upper and lower core sections are more enriched than the middle zone. In general, the values ranging from -26 to -28 are consistent with marine phytoplankton, and would be more enriched in 13C if ice algae was a major component to the organic matter preserved in the sediment cores. The measured carbon isotope shifts may represent changes in water temperature with an overall increase in temperature over the last ~400 years. Conversely, the 13C may also be reflecting changes in rates of primary productivity with higher rates corresponding to higher 13C values.
- BiSi values range from 0.5 to 7% with the highest levels peaking in the upper third of the short core and in the upper 20 cm of the meter-long vibracore with minimal values in the central section, and more moderate values at depth. Elevated BiSi values likely result from enhanced periods of primary productivity in the lake basin brought about by either an increase in temperature, or an increase in ice-free days.
A preliminary interpretation is that varve thickness, 13C and biogenic silica records indicate that Cape Hurd Lake has experienced the highest rates of primary productivity and perhaps warmest temperatures over the last 300 years. Two periods of lower productivity and colder temperatures are indicated by thinner varves, low BiSi, and low 13C occurred prior to this period, i.e. during the early phases of the Little Ice Age. Slightly higher values in the proxies for the period from ca. 1100 B.P. to 2200 B.P. indicate conditions more moderate conditions.
References cited:
ACIA, 2004, Impacts of a Warming Arctic, Arctic Climate Impact Assessment: CambridgeUniversity Press, 139 p.
Putnam, A.E., 2004, Recent sedimentation in a transect of high arctic isolation basins,southern Queen Elizabeth Islands, Nunavut, Canada, Undergraduate honors thesis, Bates College, 190 p.
Saenger, C.P., 2002, Holocene basin evolution and paleoclimate reconstruction from laminated sediments, Cape Hurd Lake, Devon Island, Nunavut, Canada, undergraduate honors thesis, Bates College, 98 p.
Stoner, J.S., Francus,P., Bradley, R.S., Patridge, W., Abbott, M., Channell, J., and Retelle, M., 2004, Late Holocene Paleomagnetic Secular Variation (PSV) from Nunavut, Canada, Abstract, Annual Arctic Workshop, Boulder, CO.
A Virtual Expedition to Swedish Arctic Research Platforms
Gunhild (Ninis) Rosqvist1
1Department of Physical Geography and Quaternary Geology, Stockholm University, Institutionen för naturgeografi och kvartärgeologi, Frescativägen 8, Stockholm, SE-106 91, Sweden, Phone +46-8-164983, Fax +46-8-164818, gunhild.rosqvist [at] natgeo.su.se
This expedition will take us to the two research stations located in arctic Sweden, Tarfala Research Station and Abisko Scientific Research Station. The glaciological, hydrological, meteorological and biological research and long-term monitoring carried out at these stations will be introduced briefly. During the IPY data showing the dynamic response of Swedish glaciers will feed into international projects and new studies of the effect of climate change on the reindeer herding has recently started. During the IPY the Kinnvika station located on Nordaustlandet, Svalbard, will be reactivated again for research in different disciplines. One project will for example focus on the coupling between the present climate change and the Vestfonna ice cap. The Swedish icebreaker Oden will travel along the Lomonosov ridge in the Arctic Ocean to map the bathymetry in detail using a multi-beam echo-sounder during the summer of 2007. The ocean circulation and the sea ice extent will also be studied. Oden will be used for studies of processes forming summer clouds in the Arctic in the summer of 2008.
Monitoring Frost Heave and Thaw Subsidence in at CALM Sites in Northern Alaska with Differential GPS
Dmitry A. Streletskiy1, Jonathon D. Little2, Nikolay I. Shiklomanov3, Frederick E. Nelson4
1Department of Geography, University of Delaware, 216 Pearson Hall, Newark, DE, 19716, USA, Fax 302-831-6654, strelets [at] udel.edu
2Department of Geography, University of Delaware, 216 Pearson Hall, Newark, DE, 19716-2541, USA, Phone 302-831-2344, Fax 302-831-6654, jlittle [at] udel.edu
3Department of Geography, University of Delaware, 216 Pearson Hall, Newark, DE, 19716, USA, Phone 302-831-1314, Fax 302-831-6654, shiklom [at] udel.edu
4Department of Geography, University of Delaware, 216 Pearson Hall, Newark, DE, 19716, USA, Phone 302-831-0852, Fax 302-831-6654, fnelson [at] udel.edu
Vertical movement of the ground surface due to frost heave and thaw subsidence is a common phenomenon in permafrost regions. The magnitude of this movement varies both temporally and spatially, owing to interannual climatic variability at the ground surface and to local variations of soil moisture. Traditional survey methods for measuring vertical surface movement have some disadvantages. Geodetic surveys have good spatial coverage, but are limited to small numbers of survey benchmarks in the Arctic. Frost tubes and heavometers allow precise measurements of heave/subsidence, but are usually limited to point locations or very small areas. Differential Global Positioning Systems (DGPS) provides an alternative approach for measuring ground heave/subsidence that can fill the gap between spatial coverage and precision. DGPS technology, in conjunction with survey targets designed specifically for this study, was employed at three sites in the northern Brooks Range Foothills and the Arctic Coastal Plain of northern Alaska. Beginning in 2001, vertical movement of the ground surface was measured twice per year (June and August). The resulting five-year heave/subsidence record, in conjunction with temperature and active-layer measurements, was used to evaluate regional and site-specific factors affecting the spatial and temporal variability of frost heave and subsidence. Results indicate that heave and settlement show patterns of spatial variation similar to those of active-layer thickness, but that progressive penetration of thaw into the ice-rich transient layer occurred between 2001 and 2006. Comparison of GPS-measured ground surface elevations with historic data reveals significant long-term ground subsidence at several sites near Barrow. DGPS methodology is useful for measuring frost heave and thaw settlement over relatively large areas with very fine resolution.
Arctic Sea Ice is Disappearing Faster than Forecast
Julienne C. Stroeve1, Mark C. Serreze2, Marika Holland3, Walter Meier4
1National Snow and Ice Data Center, University of Colorado, UCB 449, Boulder, CO, 80309-0449, USA, Phone 303-492-3584, Fax 303-492-2468, stroeve [at] kryos.colorado.edu
2National Snow and Ice Data Center, University of Colorado, UCB 449, Boulder, CO, 80309-0449, USA, Phone 303-492-2963, Fax 303-492-2468, serreze [at] kryos.colorado.edu
3Climate and Global Dynamics Division, National Center for Atmospheric Research, PO Box 3000, Boulder, CO, 80307, USA, Phone 303-497-1734, Fax 303-497-1700, mholland [at] cgd.ucar.edu
4National Snow and Ice Data Center, University of Colorado, UCB 449, Boulder, CO, 80309-0449, USA, Phone 303-492-6508, Fax 303-492-2468, walt [at] nsidc.org
From 1953 to 2006, arctic sea ice extent at the end of the melt season in September has declined sharply. All models participating in the Intergovernmental Panel on Climate Change Fourth Assessment Report (IPCC AR4) show declining arctic ice cover over this period. However, depending on the time window for analysis, none or very few individual model simulations show trends comparable to observations. Current arctic summer sea ice conditions are on average thirty years ahead of where the models say we should be. Since the IPCC models suggest summer ice-free conditions as early as 2050, this state to a seasonally ice free Arctic may occur sooner than projected.
Results from the study also suggest greenhouse gases are playing a significant role in the observed decline. If the multi-model ensemble mean time series provides a true representation of forced change by greenhouse gas (GHG) loading, 33-38% of the observed September trend from 1953-2006 is externally forced, growing to 47-57% from 1979-2006. Given evidence that as a group, the models underestimate the GHG response, the externally forced component may be larger.
Although it remains a mystery as to the exact date when the Arctic may become ice free in summer and how rapid this transition may be, this new state would have profound implications for climate around the globe. A warmer Arctic will result in altered atmospheric and oceanic circulation patterns that will affect weather worldwide. Studies have already linked arctic sea ice loss to changes in atmospheric patterns that cause reduced rainfall in the American West and increased precipitation over western and southern Europe.
Rivers, Lakes, Tundra, and Taiga: A Journey Across Alaska and Northern Canada
Matthew Sturm1
1Cold Regions Research and Engineering Laboratory - Alaska, PO Box 35170, Ft. Wainwright, AK, 99703-0170, USA, Phone 907-353-5183, Fax 907-353-5142, msturm [at] crrel.usace.army.mil
U.S. and Canadian International Polar Year (IPY) scientists drove snowmobiles from Fairbanks, Alaska to Baker Lake, Nunavut during March and April of 2007, traveling 4300 km. Their route lay along the Yukon, MacKenzie and Thelon Rivers, as well as many lesser rivers. It included a traverse of Great Bear Lake, the 8th largest lake in the world. It wound in and out of the taiga and tundra, and it took them through 11 arctic communities, most of which subsist off the land. Along the way, past, present, and future sites of mining and oil and gas extraction were examined. Across this vast area, historically the waterways were, and they still remain, crucial, to the transportation system. Water, in the form of snow and rain, is one of the primary agents in landscape evolution, and alterations in water regimes due to climate change is likely to have a more profound impact on ecosystems and humans than any changes in temperature. Vignettes from the journey will be used to illustrate these points.
Melting Over the Greenland Ice Sheet in 2006 and the 2003 - 2006 Melting Anomaly from Space-Borne Microwave Data
Marco Tedesco1
1JCET, NASA/University of Maryland Baltimore County, NASA GSFC, code 614.6, Greenbelt, MD, 20771, USA, Phone 301-614-5717, Fax 301-614-5558, mtedesco [at] umbc.edu
Greenland is the world's largest island, containing the second largest glacier on Earth (the Inland Ice) with a surface extent of approximately 1.75 million Km2. It is also the largest relic of the Ice Age in the Northern Hemisphere, containing enough ice to rise the sea level of around 7 m. Recently, the Intergovernmental Panel on Climate Change (IPCC, available at http://www.ipcc.ch/SPM2feb07.pdf) estimated contribution to the sea level rise from the Greenland ice sheet at 0.21 mm/year, representing 7.5% of the sum of individual climate contributions to sea level rise (2.8 mm/year) and 6.7% of the observed total sea level rise.
Wet and dry snow have different physical properties, although they appear to be very similar at first look. Scientists agree that the presence of liquid water within the snowpack causes more incoming solar radiation to be absorbed than dry snow. Hence, as Greenland is very sensitive to changes in the climatic forcing, a rise in the temperature will increase the areal extent of surface snowmelt. This decreases the amount of solar energy reflected back to space, which, in turn, will increase the energy absorbed by the Earth. Also, recent studies show that melting snow enhances sliding of glaciers as surface meltwater seeps down to the ice-bedrock interface. Furthermore, increased melting liberates water that can freely evaporate; this may increase cloud cover, which is associated with its own complex set of climate feedbacks.
In this poster, I will show maps of melt extent and duration in 2006 over the Greenland ice sheet derived from space-borne microwave brightness temperatures measured by the Special Sensor Microwave Imaging radiometer (SSM/I). I will also show temporal trends of daily melting areas from which distinct events regarding the onset melting and freezing can be identified. A long-term analysis for the period 1988-2006 shows that 2006 ranked seventh or fifth, depending on the frequency, within the study period. Lastly, melting anomalies for the period 2003-2006 will be displayed, showing consistent results with those regarding mass loss, altitude or surface temperature changes from other satellites mission, such as GRACE., ICESat and MODIS.
ISAC - International Study of Arctic Change
Michael Tjernström1, Grete K. Hovelsrud2
1Department of Meteorology, Stockholm University, Arrhenius Laboratory, Stockholm, SE-106 91, Sweden, Phone +46 8 163110, Fax +46 8 157185, michaelt [at] misu.su.se
2Center for International Climate and Environmental Research (CiCERO), PO Box 1129, Blindern, OSLO, N-0318 , Norway, Phone +47 22 85 87 69, Fax +47 22 85 87 51, g.k.hovelsrud [at] cicero.uio.no
The International Study of Arctic Change (ISAC) is science program jointly launched by the International Arctic Science Committee (IASC) and the Arctic Ocean Sciences Board (AOSB). It is designed as a long-term, international, cross-disciplinary and pan-arctic program to document arctic environmental changes. ISAC will take a system approach to facilitate expansion and deepening of our knowledge of the arctic system and to document changes in the Arctic. ISAC is motivated by already documented changes large enough to affect the lives of native populations, other residents in the arctic realm, as well as the ecosystem. Climate models suggest substantial future changes; these will have a profound impact on Humankind not only at high latitudes but potentially also at lower latitudes through climatic tele-connections. It is obvious that the distribution of marine sustainable resources will change, but to what degree and in what patterns is not possible to answer with certainty before reliable climate forecasting can be performed. It cannot be disregarded that poorly understood feedback mechanism might alter the system resulting in abrupt changes in climate. Whereas dealing with changes is challenging enough, the lack of predictability compounds the problem because it does not allow development of strategies to deal with the changed environment. Among the major products of ISAC will be contributions to scientific assessments that address present and future needs of impact assessments. Toward this end ISAC will engage in multidisciplinary observational, synthesis and modelling activities responding to societal needs, science plans and priorities established by scientific community.
Arctic Atmospheric Modeling: Should We Believe the Models?
Michael Tjernström1
1Department of Meteorology, Stockholm University, Arrhenius Laboratory, Stockholm, SE-106 91, Sweden, Phone +46 8 163110, Fax +46 8 157185, michaelt [at] misu.su.se
Climate models form a backbone for climate research. Not only are they the only way by which we can say something about the future, but they are also fundamental tools for understanding the climate system. The climate system is so complex and non-linear that it is basically impossible for the human mind to comprehend without the aid of models describing as much as possible of all the processes and their interactions within the system.
But what is a climate model and why should we believe in them? From a philosophical point, a climate model is the aggregate of all our understanding of how the system, and the parts thereof, work. It is the sum total of all hypotheses about the processes we understand, refined to the point possible given the uncertainty of our current knowledge and the extent to which current computing power allows an implementation of this understanding into computer code. Climate models thus contain all the things we are pretty sure about, quite a few things we have a fair but not complete understanding about but also processes were we know we could do a lot better but are hindered by the practicalities of current modeling techniques. And there are also the things we sense might be important but do not know how to formalize into equations the way a model most be designed, and - off course - all the processes that we don't know that we don't know about; these could be important or not and since we don't know, how can we make an informed judgment?
In practice, we thus tend to believe in climate models for two reasons: 1) They are capable of reconstructing present climate, given the climate forcing of today; 2) They (are supposed to) take into account all the processes we think are important, including their interactions. At the heart of this problem lies the scale separation between resolved and unresolved scales. As computing power increases, the resolved scales becomes finer and finer and today's models properly resolve the "synoptic scale", or what we commonly refer to as the "weather systems". But a paradox in climate modeling is that the processes that drives the climate - and climate change - will likely "forever" remain unresolved in these models. In the atmosphere this is the case for radiation, cloud and aerosols, precipitation, boundary-layer turbulence, the energy balance and the exchange processes at the surface. These are all processes that have to be parameterized - described as functions of the resolved scale variables, applying some understanding that ultimately always relies on empirical evidence. This implies a fundamental uncertainty, and the IPCC AR4 report also points to the cloud processes as a fundamental reason for different models having different climate sensitivity. For the Arctic this is a particular problem since the body of empirical evidence for any process is small, certainly smaller than for other regions - or climate regimes - on Earth.
In this presentation I aim to show that both the fundamental cornerstones for believing in climate models may in fact be rather shaky at best. Current global climate models do not necessarily do a very good job of reconstructing the current arctic climate. And while the relevant processes are there, they are parameterized based on empirical evidence that does not come from the Arctic and thus, due to aspects of some climate processes that are unique to the Arctic, they don't always work very well. On top of these issues are off course the large inherent climate variability of the entire arctic climate system. Thus the paradox that although climate change is the fastest in the Arctic, the tools we use to understand this change are at the same time the poorest. The challenge therefore becomes to improve the representation of arctic climate in global climate models while at the same time preserving or improving their overall performance. This can only happen if we learn more about climate processes in the Arctic, which imply in-situ process studies in the Arctic. This is what IPY is - or should - be all about.
Synoptic Influences on the Arctic's Freshwater Budget: Case Studies From the Northern North Atlantic
Maria Tsukernik1, Mark C. Serreze2, David N. Kindig3
1National Snow and Ice Data Center, University of Colorado Boulder, University Campus Box 449, Boulder, CO, 80309, USA, Phone 303-492-6115, tsukerni [at] colorado.edu
2National Snow and Ice Data Center, University of Colorado Boulder, University Campus Box 449, Boulder, CO, 80309, USA, Phone 303-492-2963, Fax 303-492-2468, serreze [at] kryos.colorado.edu
3National Snow and Ice Data Center, University of Colorado Boulder, University Campus Box 449, Boulder, CO, 80309, USA, Phone 303-492-6115, kindig [at] nsidc.org
The freshwater budget of the Arctic has drawn scientific attention for several decades. The shrinkage of sea ice observed in recent decades together with the increased high latitude storm activity and precipitation has a potential to alter the rate of thermohaline circulation in the North Atlantic and thus influence the global climate. The majority of research devoted to this problem has focused on a monthly and/or annual timescale. We compliment current research by looking at the key variables of the arctic freshwater budget on a synoptic timescale.
In addition to its significance in the global climate system, the northern North Atlantic region is one of the most synoptically active and variable regions of the planet, especially during the winter season. Through impacts on net precipitation and wind driven export of sea ice via Fram Strait, these cyclones can modulate arctic freshwater budget.
NCEP/NCAR reanalysis data are used to examine winter synoptic activity in the North Atlantic. The cyclone identification algorithm is based on the sea level pressure (SLP) pressure field. We use ERA-40 6-hourly fields to assess precipitation, evaporation and moisture flux and daily sea ice concentration from Nimbus-7 SMMR and DMSP SSM/I passive microwave and daily Polar Pathfinder Ease-Grid sea ice motion vectors to investigate sea ice, the later two are distributed at the National Snow and Ice Data Center (NSIDC). We identify several typical cases and analyze their regional precipitation and sea ice input.
Precipitation follows the cyclone activity closely. Generally, the more intense the synoptic system is, the more precipitation is associated with it. However, since cyclones vary in size and developmental patterns, case study approach proves to be the most valuable tool to assess precipitation. Cyclone generated low level wind have a huge effect on sea ice motion when the storm is fairly deep and fairly close to the sea ice margin. Using several case studies we illustrate these associations in greater detail.
The Arctic in the IPCC Fourth Assessment Report
John Walsh1
1International Arctic Research Center, University of Alaska Fairbanks, 930 Koyukuk Drive, Fairbanks, AK, 99775, USA, Phone 907-474-2677, Fax 907-474-2643, jwalsh [at] iarc.uaf.edu
The Arctic, especially its hydrology, is more prominent in the 2007 report of the Intergovernmental Panel on Climate Change (IPCC) than in previous IPCC reports. While some of the IPCC's findings about the Arctic draw upon the Arctic Climate Impact Assessment, there are notable additions and updates. Key arctic-related findings are an accelerated rate of decrease of summer sea ice (-7.4% +/- 2.4% per decade); an acceleration of the total cryospheric contribution to sea level change from 0.2-0.4 mm/year to about 1 mm/yr in the past five to ten years; warming of the upper permafrost layers by up to 3°C since the 1980s; a reduction in the maximum area of seasonally frozen ground by 7% (15% in spring); and a recent freshening of subarctic seas.
Among the impacts that have been identified (with "high confidence" or better) are changes in species' ranges and abundances, and in the positions of some tree lines in the Arctic; improved marine access, increased coastal wave action, changes in coastal ecology, including adverse impacts on ice-dependent marine wildlife; and indications that Arctic Indigenous Peoples are already adapting to climate change but facing a combination of stressors that are severely testing their resiliency.
Projected changes for the 21st century include a warming of 4-5°C, largest in the autumn and winter and over the polar oceans; increases of precipitation and especially the rain-to-snow ratio; increases of river discharge by 10-30%; thawing of some areas of discontinuous permafrost, and a general thickening of the active layer by 30-50% by 2100, leading to destabilization of river banks and slopes, increased erosion and sediment supply; and increased discharge from glaciers and ice sheets Positive impacts of the projected climate change include reduced heating costs, increased agricultural and forestry opportunities, and improved marine access to the Arctic. Major uncertainties are the future rates of ice mass loss from Greenland and the rates of permafrost degradation.
IPY: Impacts of Permafrost Degradation on Methane Emissions From Arctic Lakes
Katey M. Walter1, Mary E. Edwards2, Guido Grosse3, F. Stuart Chapin III4, Sergey Zimov5, Sudipta Sarkar6, Laurence C. Smith7, Lawrence Plug8
1Institute of Northern Engineering, University of Alaska Fairbanks, Water and Environmental Research Center, Duckering Building, Fairbanks, AK, 99775, USA, Phone 907-474-6095, Fax 907-474-6967, ftkmw1 [at] uaf.edu
2School of Geography, University of Southampton, Highfield, Southampton, -, SO17 1BJ, UK, Phone +(44)(0)2380592, Fax +44 (0)23805932, M.E.Edwards [at] soton.ac.uk
3Geophysical Institute, University of Alaska Fairbanks, Fairbanks, AK, 99775, USA, Phone 907-474-7548, ggrosse [at] gi.alaska.edu
4Institute of Arctic Biology, University of Alaska Fairbanks, PO Box 756100, Fairbanks, AK, 99775-6100, USA, Phone 907-474-7922, Fax 907-474-6967, fffsc [at] aurora.uaf.edu
5Northeast Science Station, Russian Academy of Sciences, PO Box 18, Republic Sakha - Yakutiya, Cherskii, -, 678830, Russia, Phone +7-41157-23013, Fax +7-41157-22560, sazimov [at] cher.sakha.ru
6Geophysical Institute, University of Alaska Fairbanks, Fairbanks, AK, 99775, USA, Phone 907-474-6839, ftss4 [at] uaf.edu
7Department of Geography, University of California Los Angeles (UCLA), PO Box 951524, 1255 Bunche Hall, Los Angeles, CA, 90095-1524, USA, Phone 310-825-3154, Fax 310-206-5976, lsmith [at] geog.ucla.edu
8Department of Earth Sciences, Dalhousie University, Life Sciences Centre, Oxford Street, Halifax, NS, B3H 4J1, Canada, Phone 902-494-1200, lplug [at] dal.ca
A recent first-order estimate suggests that arctic lakes are significant emitters of methane (CH4) contributing as much as ~6% of global atmospheric CH4 sources annually. Emissions from arctic lakes are projected to increase as permafrost thaws in the Arctic, releasing tens of thousands of teragrams to the atmosphere in the form of bubbles. Emissions are particularly high from lakes influenced by permafrost degradation, a process that discharges labile organic matter to anaerobic lake bottoms, fueling biological methane production and emissions. Complete thaw of permafrost beneath lakes may destabilize deeper methane sources such as hydrate methane and provide pathways for the release of sub-permafrost methane from biogenic or thermogenic sources. Despite the potential for catastrophic release of deep methane pools to the atmosphere, little is known about the occurrence, extent, and vulnerability of these methane sources. Quantifying, mapping, and projecting biological and geological methane emissions from arctic lakes in Alaska and Siberia in conjunction with permafrost degradation are the goals of our research group during the IPY by pioneering new methods of measuring methane bubbling (dominant mode of emissions) from lakes using geophysical measurements, isotope geochemistry, remote sensing and the establishment of a Pan-Arctic Lake-Ice Methane Monitoring Network (PALIMMN). We aim to link paleo-records of thaw lake emissions to earth-system modeling to improve understanding of the role of thaw lakes in climate change over millennial time scales and to project emissions from arctic lakes in the future as permafrost continues to warm and thaw. This collaborative effort involves a highly interdisciplinary and international team of researchers from the United States, Canada, United Kingdom, India, Russia, Germany, Sweden, and Romania as well as integration with IPY certified projects including SEARCH, AON: Collaborative Research on Carbon, Water, and Energy Balance of the Arctic Landscape at Flagship Observatories and in a Pan-Arctic Network, Permafrost Observatories: Thermal State of Permafrost, Arctic Circum-Polar Coastal Observatory Network, and Carbon Pools in Permafrost Regions.
Vulnerability to Changing Conditions in the Canadian Arctic: The Case of Arctic Bay, Nunavut
Johanna Wandel1
1Department of Geography, University of Guelph, Global Environmental Change Group, Guelph, ON, N1G 2W1, Canada, Phone 519-824-4120, Fax 519-837-0811, jwandel [at] uoguelph.ca
This paper outlines a methodological framework employed in a number of empirical analyses aimed at identifying vulnerability to changing environmental conditions in human communities. The approach involves categorizing current and past exposure-sensitivities and adaptive strategies from a variety of sources and drawing on these to identify implications and possible adaptive strategies related to anticipated changes in environmental conditions relevant to the community. The approach is illustrated with a case study from the community of Arctic Bay, in Canada's High Arctic. The framework forms the basis for comparison and integration among a number of case studies currently being undertaken as part of International Polar Year.
The Arctic Research Consortium of the United States
Wendy K. Warnick1
1Executive Director, ARCUS, 3535 College Road, Suite 101, Fairbanks, AK, 99701, USA, Phone 907-474-1600, Fax 907-474-1604, info [at] arcus.org
The Arctic Research Consortium of the United States (ARCUS) is a nonprofit membership organization, composed of universities and institutions that have a substantial commitment to research in the Arctic. ARCUS promotes arctic research by improving communication among the arctic research community, by organizing workshops, and by publishing scientific research plans. ARCUS was formed in 1988 to serve as a forum for planning, facilitating, coordinating, and implementing interdisciplinary studies of the Arctic; to act as a synthesizer and disseminator of scientific information on arctic research; and to educate scientists and the general public about the needs and opportunities for research in the Arctic.
Effects of Changes in Water Resources on Northern Societies
Daniel M. White1, Larry D. Hinzman2, Liliam Alessa3, Andrew Kliskey4, Peter P. Schweitzer5
1Institute of Northern Engineering, University of Alaska Fairbanks, PO Box 755900, Fairbanks, AK, 99775-5900, USA, Phone 907-474-6222, Fax 907-474-6866, ffdmw [at] uaf.edu
2International Arctic Research Center, University of Alaska Fairbanks, PO Box 757340, Fairbanks, AK, 99775-7340, USA, Phone 907-474-7331, Fax 907-474-1578, ffldh [at] uaf.edu
3Resilience and Adaptive Management Group , University of Alaska Anchorage , 3211 Providence Drive , Anchorage , AK, 99508, USA, Phone 907-786-1507, Fax 907-786-7749, afla [at] uaa.alaska.edu
4Department of Biological Science, University of Alaska Anchorage, 3211 Providence Drive, Anchorage, AK, 99508, USA, Phone 907-786-1136, Fax 907-786-4607, afadk [at] uaa.alaska.edu
5Department of Anthropology, University of Alaska Fairbanks, PO Box 757720, Fairbanks, AK, 99775-7720, USA, Phone 907-474-5015, Fax 907-474-7453, ffpps [at] uaf.edu
Freshwater is critical to the sustainability of people and their activities in the Arctic. The availability and status of water resources may promote good health or propagate disease, support the distribution and quality of plants and animals used for subsistence, and promote or impede transportation and resource development. Water is integral to the culture of arctic people. In the past 30 years, the climate in the Arctic has warmed appreciably and there is evidence for a significant polar amplification of global warming in the future. Recent studies suggest that climate change is having, and will continue to have a significant impact on arctic hydrology and Northern societies. For example, of concern to people in the Arctic are lakes disappearing with the loss of permafrost, low river stage limiting access to subsistence resources, and the availability of water for construction of ice roads. This presentation will discuss how humans depend on freshwater at local scales in the Arctic, and how climate change is affecting this dependency.
Community Needs Assessment and Portal Prototype Development for an Arctic Spatial Data Infrastructure (ASDI)
Helen V. Wiggins1, Wendy K. Warnick2, Lamont C. Hempel3, Jordan Henk4, Mark Sorensen5, Craig E. Tweedie6, Allison Graves7
1Program Coordinator, ARCUS, 3535 College Road, Suite 101, Fairbanks, AK, 99709, USA, Phone 907-474-1600, Fax 907-474-1604, helen [at] arcus.org
2Executive Director, ARCUS, 3535 College Road, Suite 101, Fairbanks, AK, 99709-3710, USA, Phone 907-474-1600, Fax 907-474-1604, warnick [at] arcus.org
3The Redlands Institute, University of Redlands, PO Box 3080, 1200 E. Colton Avenue, Redlands, CA, 92373, USA, Phone 909-793-2121, monty_hempel [at] redlands.edu
4The Redlands Institute, University of Redlands, PO Box 3080, 1200 E. Colton Avenue, Redlands, CA, 92373, USA, Phone 909-793-2121, jordan_henk [at] redlands.edu
5Redlands Institute, University of Redlands, PO Box 3080, 1200 E. Colton Avenue, Redlands, CA, 92737, USA, Phone 909-867-7628, Fax 909-867-5310, mark_sorensen [at] redlands.edu
6Department of Biology, University of Texas at El Paso (UTEP), 500 West University Avenue, El Paso, TX, 79968-0513, USA, Phone 915-747-8448, Fax 915-747-5808, ctweedie [at] utep.edu
7Nuna Technologies, PO Box 1483, Homer, AK, 99603, USA, Phone 907-235-3476, nunatech [at] usa.net
As the creation and use of geospatial data in research, management, logistics, and education applications has proliferated, there is now a tremendous potential for advancing science through a variety of cyberinfrastructure applications, including Spatial Data Infrastructure (SDI) and related technologies. SDIs provide a necessary and common framework of standards, securities, policies, procedures, and technology to support the effective acquisition, coordination, dissemination and use of geospatial data by multiple and distributed stakeholder and user groups. Despite the numerous research activities in the Arctic, there is no established SDI and, because of this lack of a coordinated infrastructure, there is inefficiency, duplication of effort, and reduced data quality and search ability of arctic geospatial data. The urgency for establishing this framework is significant considering the myriad of data that is likely to be collected in celebration of the International Polar Year (IPY) in 2007-2008 and the current international momentum for an improved and integrated circumarctic terrestrial-marine-atmospheric environmental observatories network. The key objective of this project is to lay the foundation for full implementation of an Arctic Spatial Data Infrastructure (ASDI) through an assessment of community needs, readiness, and resources and through the development of a prototype web mapping portal.